RISK FACTORS FOR PROGRESSIVE CKD
The effort to reduce CKD began with therapy for proteinuria and hypertension, which are recognized risk factors for CKD.54,55 The rate of progressive renal deterioration has a linear relationship with blood pressure treated by anti-hypertensive agents.56 Large controlled trials have documented the protein reduction properties of effective anti-hypertensive therapy.57–59 The success in implementing these therapies led to the identification of other CKD risk factors.
Proteinuria The relationship between reduced proteinuria and progressive renal disease was first demonstrated in the Modification of Diet in Renal Disease (MDRD) Study in 1995, 55 which also showed that patients with high urinary protein excretion benefit more from ACE inhibitor-based therapies. 55 The inhibition of the RAAS either via ACE inhibitors or angiotensin receptor blockers (ARBs) reduces proteinuria and progressive renal deterioration in excess of what would be expected based on the reduction of blood pressure alone with other non-RAAS agents. 60 The ACE Inhibition in Progressive Renal Disease (AIPRD) study, a cumulative meta-analysis of 11 clinical trials including the Ramipril Efficacy in Nephropathy (REIN) study, found a strong correlation between proteinuria and the decline rate of glomerular filtration rate (GFR) in patients with CKD. 61 Together, the MDRD and AIPRD studies revealed an impressive 40% reduction in the risk of doubling serum creatinine concentrations with ACE inhibitor treatment compared with other antihypertensive drugs in patients with CKD with protein levels >0.5 g per day. 62 A similar effect was shown in patients with type 1 diabetes undergoing captopril treatment. 63
The Reduction of End Points in NIDDM with the Angiotensin II Receptor Antagonist Losartan (RENAAL) study found that baseline albuminuria was the strongest predictor of the primary composite end-point of doubling of serum creatinine, end-stage renal disease (ESRD), or death in patients with type 2 diabetes mellitus (T2DM) with a serum creatinine level of 1.5–3.0 mg/dL.34 Overt proteinuria or microalbuminuria predicted kidney deterioration in a population with a high prevalence of CKD,64 individuals with hypertension and diabetes, and the general population.29,65,66 Significantly lower persistent microalbuminuria (6% in 3 years) was observed in patients with hypertension and T2DM with normal albuminuria treated by trandolapril compared to those receiving placebo (10%) or verapamil (11.9%).67 The anti-proteinuric and renal protection provided by ACE inhibitors was also observed in patients with diabetes and normal blood pressure but without microalbuminuria. The increase of albuminuria, even within the normal range, and the decrease of creatinine clearance were significantly lower in patients receiving enalapril compared with those receiving a placebo.68
The theoretical added therapeutic benefit of ARBs emanates from the blockage of angiotensin II to the angiotensin type 1 (ATI) receptor interactions as well as through enhanced angiotensin II binding to the vasodilatory angiotensin type 2 (ATII) receptors.69 Albuminuria decreased by 28% among losartan-treated patients over the first 6 months of the RENAAL study compared with a 4% increase among the placebo group. The decrease in albuminuria in the losartan group was associated with improved kidney function, going beyond the drug’s blood pressure-lowering effect.34 Changes in albuminuria showed an approximately linear relationship with the degree of long-term kidney protection. In particular, every 50% reduction in albuminuria was associated with a corresponding ~36% reduction in the risks of doubling of serum creatinine level, stage 5 CKD, or death. A similar effect was observed regarding other ARBs32,70 such as irbisartan. Treatment with ARBs has also been successful in patients with incipient diabetic nephropathy.71,72 The anti-albuminuric and renal protective effects of ARBs are similar, although slightly weaker, than the corresponding effects of the ACE inhibitors in early diabetic nephropathy.73
Despite the enormous progress that has been made in the treatment of progressive kidney disease via RAAS inhibition, the residual kidney risk after treatment with an ACE inhibitor or an ARB remains high and is associated with residual albuminuria.74,75 For example, in the RENAAL trial, losartan reduced the 3-year risk of doubling serum creatinine levels from 47% to 44%.33 In light of these high residual risk rates, recent reviews have examined various new strategies to enhance the effects of RAAS blockade.69,76 The RAAS is an endocrine cascade system that can be inhibited at many levels, but it can be compensated for at other levels with a clinical response known as “escape.”69,76
Clinical trials have examined the use of the combination of an ACE inhibitor and an ARB to prevent target organ damage.77 The Renal Outcomes with Telmisartan, Ramipril, or Both in People at High Vascular Risk (ONTARGET) trial compared the ACE inhibitor ramipril with the ARB telmisartan, alone and in combination, among patients at high risk for vascular disease.78,79 Although the achieved mean blood pressure was lower in patients who received telmisartan or both agents than in those who received ramipril alone, no difference was observed with regard to the primary outcomes among any of the groups, and more adverse outcomes were noted in the combination group. Importantly, this trial did not evaluate ARB and ACE inhibitor therapy in patients with advanced proteinuric renal disease. The VA Nephron-D Diabetes in Nephropathy Study (VA NEPHRON-D), a trial using a combination therapy (i.e. ACE inhibitor and ARB therapy versus ARB monotherapy) in patients with proteinuric diabetic nephropathy, was stopped because of the increased adverse events of hyperkalemia and acute kidney injury (AKI).80 The Aliskiren Trial in Type II Diabetes Using Cardiorenal Endpoints (ALTITUDE) randomly assigned 8,561 patients to aliskiren (300 mg daily) or a placebo as an adjunct to ACE/ARB monotherapy as an angiotensin receptor blocker. The trial was stopped prematurely because of adverse events (hyperkalemia and hypotension).81 Therefore, ACE inhibitors should not be used concomitantly with ARBs and renin inhibitors because of the increased risks for hypotension, hyperkalemia, and renal dysfunction.82
Many studies have attempted to achieve additional benefit from ACE inhibitors and other renin–angiotensin–aldosterone-blocking agents by increasing their dosages. This reasoning is based on the original observation that the optimal anti-proteinuric dose is not necessarily equal to the optimal antihypertensive dose. Many of these results have shown additional proteinuria reduction,83–87 whereas others have not.88–90 However, similar to the initial studies regarding combination therapy with renin–angiotensin–aldosterone-blocking agents, many of these high-dosage studies are also short-term examinations using blood pressure and albuminuria as outcome variables. These studies have not had sufficient power, and lack the duration needed to detect the safety signals and side effects rates that might emerge from end-point trials.82 Therefore, before ultrahigh RAAS-blocking agent dosing can be recommended as a renoprotective therapy, further study of these drugs with kidney and cardiovascular event data is needed.82
Optimization strategies for RAAS blockade have been suggested. First, a combination of sodium restriction and diuretic therapy is required to reach optimal RAAS inhibition in proteinuric patients.91–96 Second, hyperkalemia, which limits the use of RAAS agents, has recently received effective treatment with patiromer and sodium zirconium cyclosilicate among outpatients.97,98 These two novel drugs add to the pharmacopoeia that until recently was limited to sodium and calcium polystyrene sulfonate, which have adverse gastrointestinal effects.
Importantly, a few clinical caveats exist when treating proteinuria with RAAS inhibitors. First, greater initial decreases of renal function predict longer preservation of renal function.99 An initial loss of estimated GFR is not a concern unless it exceeds 30%, at which point diuretic-induced hypo-volemia and renal artery stenosis should be considered.100 In addition, the importance of monitoring urinary albumin decreases following RAAS blockade.101 If the urinary albumin–creatinine ratio is not lowered by ≥30% or to <300 mg/g despite a blood pressure lower than 130/80 combined with a low-sodium diet, then switching to another RAAS blocker or diltiazem should be considered.79,102 Greater reductions in proteinuria are seen with treatment using non-dihydropyridine calcium channel blockers (CCBs) than with dihydropyridine CCBs.102
RAAS Inhibitors for Cardiovascular Protection The ACE inhibitors reduce the rates of death, myocardial infarction, stroke, and heart failure among patients with heart failure, 103 left ventricular dysfunction, 104 previous vascular disease, 105 and/or high-risk diabetes. 106 The ARBs are an alternative for patients who cannot tolerate ACE inhibitors Although ACE inhibitors and ARBs have an additive effect, the more effective indication is to combine ACE inhibitor therapy with an aldosterone antagonist.
The MDRD study and other clinical interventions demonstrated strong interactions among proteinuria, hypoalbuminemia, blood pressure, CKD progression, and an increase in the inflammatory state.55,107 Furthermore, microalbuminuria is an independent risk factor for cardiovascular disease. In patients with T2DM, an albuminuria level of 20.1–30 mg/d was associated with a relative risk for cardiovascular disease of 9.8, and the relative risk for microalbuminuria was 12.4 compared with patients with albuminuria levels below 10 mg/d.108 The beneficial effect of albuminuria reduction for cardiovascular outcomes is likely associated with improvements in endothelial function in addition to the indirect effect mediated through the mitigation of renal dysfunction.
The ONTARGET trial assessed cardiovascular morbidity in patients with cardiovascular disease or high-risk diabetes but without significant albuminuria. Similar beneficial effects were observed regarding ARBs as ACE inhibitors for cardioprotection. However, the combination of these agents with ACE inhibitors was not associated with an increase in cardiac benefit, whereas adverse events were more common.79
Hypertension Hypertension is an uncontrolled and global public health challenge that is equally prevalent in developed and developing nations. 109 In 2000, 25% of the world’s population had hypertension; however, approximately 29% (1.56 billion people) are expected to have hypertension by 2025. This increase has been ascribed to the massive “epidemiologic transition” of the developing world, with increasing proportions of elderly populations. 110,111 Hypertension is the leading cause of cardiovascular morbidity and mortality and a major cause of CKD.
Perry et al. were one of the earliest groups to document carefully the association between increasing levels of systolic blood pressure, cardiovascular disease, and CKD risk.112 They described the direct association between increments in blood pressure elevation and the development of renal failure in 11,912 male veterans, 48% of whom were African-Americans, followed for 15 years at Veterans Administration Hypertension clinics during the mid-1970s. The risk ratios for a systolic blood pressure of 165–180 mmHg and of >180 mmHg were 2.8 and 7.6, respectively. Hospitalization for myocardial infarction doubled the risk for this disease; congestive heart failure increased the risk 5-fold and increased the rate of subsequent ESRD. The ESRD rate decreased by two-thirds among individuals whose systolic blood pressure fell by 20 mmHg.112 In addition, an increased risk of ESRD was associated with African ancestry (risk ratio=2.2).
The Multiple Risk Factor Intervention Trial (MRFIT) examined the development of cardiovascular complications in 12,000 men over 16 years and found that elevations in baseline systolic blood pressure were correlated with the development of ESRD, even within the high-normal and mild hypertensive ranges.113 This study also showed that effective blood pressure control stabilized or improved kidney function in Caucasians but not in African-Americans.114 In a 25-year observational study of 177,570 men and women, Hsu et al. demonstrated that small increases in systolic blood pressure within the pre-hypertensive and mild hypertensive ranges were correlated with increased CKD risk over time and an increase in the number of patients with ESRD.115 One risk factor for ESRD was high blood pressure (hazard ratio (HR) 2.94, 95% CI 2.21–3.92 for stage 2 hypertension; HR 2.33, 95% CI 1.78–3.05 for stage 1 hypertension; and HR 1.72, 95% CI 1.32–2.24 for pre-hypertension versus normal).
Forman and Brenner reviewed the evidence regarding a response to aggressive blood pressure reduction in “normotensive” individuals at high risk (diabetes, coronary artery disease, and cerebrovascular disease) and suggested maintaining a blood pressure below 120/80 in these patients.116 However, the clinical trials such as the ACCORD study,117 the Irbesartan Diabetic Nephropathy Trial (IDNT),118 and the International Verapamil SR-Trandolaptil Study (INVEST)119 found no benefit in bringing the blood below 130/80. Tight control of systolic blood pressure in the latter two studies did not yield improved cardiovascular outcomes and was in fact associated with an increase in all-cause mortality. These results have been summarized in other papers.120,121
Whether kidney and cardiovascular risks are lower in non-diabetic patients with CKD and blood pressures <130/80 compared with <140/90 remains unclear. This issue was examined by four randomized trials,56,59,122,123 two of which failed to show a significant benefit.59,122 However, the MDRD study showed that a reduction in blood pressure from <140/90 to <125/75 reduced the risk of kidney disease progression (HR 0.68) after 10 years of reduced blood pressure.56 Similarly, strict mean arterial blood pressure control in children below the 50th percentile for age versus a conventional therapy that corresponded to the 50th to 90th percentile for age led to decreased proteinuria and progression to ESRD (HR 0.65).124 The Cardio-Sis trial also demonstrated a benefit of blood pressure control in non-diabetic patients. Patients in the tight control group (<130 mmHg) developed less left ventricular hypertrophy (11% of 483 patients; odds ratio 0.63) and less frequently (4.8%) reached a composite cardiovascular end-point (HR 0.50) compared with patients under standard control (<140 mmHg; 17% and 9.4%, respectively).123
No evidence supports a preference for RAAS agents over other anti-hypertensive drugs among patients with CKD who present with hypertensive nephrosclerosis without proteinuria. The Antihypertensive and Lipid-Lowering Treatment to Prevent Heart Attack Trial (ALLHAT) did not find differences in the risk of exacerbated GFR or ESRD between patients given lisinopril, amlodipine, or chlorthalidone,125 even for the subgroup of patients with estimated GFRs <60 mL/min. Although proteinuria was not directly measured in these patients, it was not expected to be elevated.126
Multidrug Remission CKD Clinic Protocols Small annual differences in the rates of GFR decline can result in large differences regarding ESRD onset time. 127 The goal of the RAAS-based individually tailored multidrug anti-proteinuric and antihypertensive treatments used over the last 15 years is to reduce proteinuria and the annual decline in eGFR. 128 These protocols 129 employ a combination therapy of ACE inhibitors and ARBs shown to reduce protein, kidney, and cardiovascular events more effectively than ACE inhibitors or ARB monotherapy. 127,129,130 When proteinuria is minimal, a dual RAAS inhibitor is no more effective than a monotherapy (e.g. the ONTARGET Trial). 79 The recent closure of the ALTITUDE and VA NEPHRON-D trials has placed the use of combination RAAS therapies on hold. 80,81 A modified therapeutic strategy featuring a combination of lower-than-recommended doses of ACE inhibitors and ARB might block the RAAS system without excessive blood pressure reduction; moreover, the side effects of hyperkalemia and reduced kidney function are presently being investigated. 130 The ongoing VALID trial, Preventing ESRD in Overt Nephropathy of Type 2 Diabetes Trial, is testing whether halved dosages of ACE inhibitor and ARB administered together compared with full doses of each agent alone result in a larger reduction in proteinuria and a delay in ESRD among approximately 100 individuals with type 2 diabetes over 3 years (ClinicalTrials.gov.NCT00494715). The trial will be completed in February 2016.
Updating the “Trade-off” Hypothesis
Hyperparathyroidism. The progression of CKD and cardiovascular mortality have been directly correlated with changes in the levels of phosphate, calcium, PTH, vitamin D, and fibroblast growth factor 23 (FGF23).131 Laboratory studies in rats have demonstrated that PTH decreases glomerular filtration by decreasing the Kf on the renal podocyte.132 The suppression of PTH via parathyroidectomy, calcimimetics (calcium-sensing receptor agonists), or dietary phosphate restriction attenuated the increase in serum creatinine in a rat remnant kidney model.133 Patients with CKD and secondary hyperparathyroidism have an increased mortality risk134 and a significantly shorter renal survival than those with CKD alone.135 Parathyroidectomy effectively reduces cardiovascular events and mortality in patients receiving hemodialysis with secondary hyperparathyroidism.136
Phosphate. The effect of phosphate on CKD progression might be directly mediated by changes in renal perfusion, calcifications, and intracellular calcium-phosphate concentrations, or through its indirect effects on PTH or calcium levels.137,138 The Irbesartan Diabetic Nephropathy Trial found that the risk of doubling serum creatinine levels, ESRD, or death139 was higher by a factor of 1.8 in hyperphosphatemic diabetics.139 The AASK analysis of African-American patients with hypertensive nephrosclerosis noted that phosphorus was directly associated with a renal composite consisting of 50%, 25 mL/min GFR decline, or ESRD.140 Additional studies have shown that increasing serum phosphate concentrations are correlated with progressive renal failure141–144 and that phosphate restriction145,146 and phosphate binders stabilize renal function.147,148 An analysis of the medical files of 40,538 outpatients receiving hemodialysis registered in the Patient Profile System of Fresenius Medical Care found that high phosphorus was correlated with increased relative risks of death (1.07, 1.25, 1.43, 1.67, and 2.02 for serum phosphorus levels of 5–6, 6–7, 7–8, 8–9, and >9 mg/dL, respectively). Higher adjusted calcium levels as well as moderate and severe hyperparathyroidism (PTH levels ≥600 pg/mL) were also associated with increased rates of death.149 High phosphate is also associated with increased mortality in patients with CKD.141,149,150 Serum phosphate levels within the normal range are associated with coronary artery calcification as determined by CT scanning in patients with stage 3 and 4 CKD with or without diabetes mellitus.151 The graded152,153 associations between serum phosphate levels of >3.5 mg/dL and coronary artery calcifications,156 cardiovascular disease, and mortality have also been extended to the general population.155–159
In studying the population disparities in mineral metabolism,41,160 African-American patients with CKD demonstrate marked deficiencies in serum 25-hydroxyvitamin D (25-OH vitamin D) and higher PTH levels than Caucasians.161–163 As these patients progress toward the need for dialysis, they show even more severe secondary hyperparathyroidism and 25-OH vitamin D deficiencies.164–166 This result was also shown by the multicenter Study to Evaluate Early Kidney Disease (SEEK) in 1,860 patients with early CKD, of whom 12% were African-American. African-Americans had significantly higher PTH, calcium, phosphorus, and bone-specific alkaline phosphatase levels. In addition, they had a 1.8-fold greater risk of elevated phosphate, a 2.7-fold greater risk of a 25-OH vitamin D deficiency <30 mg/mL, and a 4.7-fold greater risk of a severe 25-OH vitamin D deficiency. They also developed secondary hyperparathyroidism earlier in their CKD course at a GFR of 45–60 mL/min, whereas Caucasians generally developed hyperparathyroidism after their GFRs decreased to <30 mL/min.167 Gutierrez et al. showed that both healthy African-Americans and those with CKD had a fractional excretion of inorganic phosphate that was approximately 30% lower than that for Caucasians (P<0.001), and the fractional excretion of calcium in African-Americans was approximately 35% lower than in Caucasians. Both African-American and Caucasian patients with CKD had eGFRs between 15 and 60 mL/min, and they had similar PTH and FGF23 levels.168
Kestenbaum and colleagues described the results of a genome-wide association study (GWAS) that investigated common genetic variations associated with serum phosphorus concentrations in the general population.169 Seven loci were described, and one locus was found directly adjacent to SLC34A1, which encodes the kidney-specific type IIa sodium-phosphate cotransporter (NaPi2a). Another was located adjacent to the calcium-sensing receptor, and one was located close to the FGF23 receptor. The SLC34A1 was also one of the 13 loci identified by the CKDGen consortium, which performed a meta-analysis of the GWAS data in 67,093 individuals of European ancestry from 20 predominate population studies to identify new genetic susceptibility loci for reduced renal function.170 Although studied extensively in murine models where NaPi2a has been shown to serve as the central mediator of renal phosphate reabsorption,171,172 the role that this transporter plays in humans had been controversial until recently. Magen and associates recently described two siblings with autosomal recessive Fanconi’s syndrome and hypophosphatemic rickets who featured a 21-base-pair in-frame duplication on SLC34A1. Functional studies have shown a complete loss of function of the mutant cotransporter, its failure to reach the plasma membrane, and an impairment of renal phosphate reabsorption. This study provided the first evidence in humans of the critical role that NaPi2a plays in human renal phosphate handling.173 Subsequently, novel loss-of-function mutations in SLC34A1 were identified in members of families with idiopathic infantile hypercalcemia (IIH) not attributed to abnormalities in inactivation of vitamin D processsing, many with nephrocalcinosis.174,175
A gain-of-function mechanism might explain the hyperphosphatemia in patients with CKD, especially in light of its recent identification as a prominent CKD locus.176 People who tend toward lower fractional excretions of phosphate might exhibit increased levels of NaPi2a.167 The high and inducible levels of expression suggest that variant versions differ in folding and may trigger an “endoplasmic reticulum associated stress response (ERAD).”177
Vitamin D. The diminished production of 1,25-OH vitamin D in renal disease likely facilitates interstitial fibrosis by allowing fibroblasts to proliferate.139 Vitamin D has been shown to prevent glomerular disease in animal models,178,179 and its derivatives decrease urine albumin excretion as well as reducing serum creatinine and glomerulosclerosis in subtotally nephrectomized rats.180,181 In a retrospective analysis, patients with CKD who were treated with calcitriol showed a decreased rate of CKD progression.182 Increasing evidence has shown that the vitamin D analogue, paricalcitol, an inhibitor of the renin–angiotensin system,183 reduces urinary albumin. Three recent studies showed that paricalcitol reduces proteinuria in patients with CKD, including those presenting with diabetes.184–186 Paricalcitol reduced albuminuria and slowed the progression of kidney injury in laboratory animals.187,188 A recent double-blind, placebo-controlled study resulted in a 20% reduction in the urinary albumin-to-creatinine ratios (P=0.053) and a 28% reduction in the 24-hour urine albumin (P=0.009) of patients receiving 2 μg of paricalcitol for 24 weeks compared with those receiving a placebo.189
Decreased levels of 25-OH and 1,25-OH vitamin D are commonly observed in patients with CKD190 as well as associated with increased cardiovascular mortality.164,191 The treatment of CKD and ESRD populations using vitamin D compounds is associated with decreased mortality rates.192,193 However, a meta-analysis of 76 trials including 3,667 participants found that these compounds failed to reduce PTH levels or mortality rates consistently.194 Newer vitamin D compounds did decrease PTH levels (by 11 pmol/L); intravenous therapy was more effective than oral therapy, but mortality rates were not affected. Additional observational studies by the same group confirmed the reduction of serum PTH and the increase in calcium and phosphorus following treatment with vitamin D compounds but failed to show increased survival rates.195,196 These studies sparked a call for randomized controlled trials to establish a causal association between vitamin D supplementation and decreased CKD mortality.197
Although the decreased production of 1,25-OH vitamin D has traditionally been ascribed to decreased renal mass (which subsequently leads to elevated serum phosphate and the inhibition of reduced 25-OH D-1alpha-hydroxylase), these mechanisms fail to explain the decline in 1,25-OH vitamin D in patients with early CKD who still have sufficient kidney mass and normal serum phosphate levels.198
FGF23. Fibroblast growth factor 23 (FGF23), which was initially characterized in a study of rare inherited disorders associated with phosphate metabolism,199 regulates phosphate homeostasis and explains the decreases in 1,25-OH vitamin D in patients with early kidney disease. Its phosphaturic effect in the proximal tubules is accomplished through the down-regulation of sodium-phosphate co-transporters, and it decreases 1,25-OH vitamin D levels via the inhibition of 25-OHD-1-alphahydroxylase and the upregulation of the 25-OHD-24 hydroxylase pathway (Figure 4).198–203 Levels of FGF23 predict CKD progression from mild to moderate in patients of European ancestry,142 and they are among the strongest markers of CKD progression, with areas under the receiver-operating characteristic (ROC) curves of 0.84 for the C-terminal FGF23 and 0.81 for intact FGF23.131 Elevated FGF23 levels in patients with early CKD also predict early cardio-vascular events such as myocardial infarction and stroke as well as the need for coronary artery or carotid artery intervention, peripheral arterial amputation or intervention, and death.204 Elevated FGF23 levels are associated with increased mortality,205,206 vascular calcifications,207 left ventricular hypertrophy and mass index,208 and bone metabolism abnormalities in patients with ESRD.209 Strategies to reduce FGF23 in early-stage CKD patients include dietary phosphate restriction,210 the use of phosphate binders,211 the administration of niacin,212 and the restriction of administration of vitamin D-type drugs and favoring therapy with calcimimetics.213,214 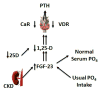 | Figure 4 The Pathogenesis of Secondary Hyperparathyroidism (SHPT) in Chronic Kidney Disease (CKD) |
The phosphate-regulating properties of FGF23 are mediated via FGFR1c, which requires alpha Klotho as a co-receptor. Its sites of action in the kidney are the subject of an active investigation and include the decreased expression of NaPi2a and NaPi2c.215 The recent demonstration of FGF23-mediated signaling in the distal convoluted tubule at the site of the alpha Klotho co-receptor adjacent to the NaPi2a-expressing proximal tubular cells likely represents FGF23 bioactivity through nephron-specific events that have yet to be elucidated.216 Saito et al. hypothesized that the initial aberration in this signaling pathway is the inappropriate upregulation of NaPi2a receptors;217 this theory is consistent with the association between the SLC34A1 locus and CKD described above.
An intriguing study recently found associations between FGF23 and body mass index (BMI), waist circumference, waist-to-hip ratio, serum lipids, and fat mass. In two cohorts of elderly European Caucasian participants, FGF23 was negatively associated with HDL and apolipoprotein A1 as well as positively associated with triglycerides. An increase of one standard deviation in the log-FGF23 levels was associated with a 7%–20% increase in BMI, waist circumference, and waist-to-hip ratio as well as a 7%–18% increase in trunk and total body fat mass as determined using whole-body dual X-ray absorptiometry. Levels of FGF23 were higher in participants with metabolic syndrome or at an increased risk of metabolic syndrome,218 indicating that FGF23 underlies cardiovascular risk via either phosphate or adverse lipid metabolism. The authors of that study cautioned against extending this association to African-American and Latino populations receiving dialysis; however, they emphasized that these populations have lower FGF23 levels205 and a dialysis survival advantage.164,219,220
Consistent experimental and human epidemiologic findings have suggested a need to test therapeutic approaches to lower phosphate levels in patients with CKD.221 Pilot studies of patients with stage 3 or 4 CKD suggest that phosphate binders, low phosphate diets, and vitamin B3 derivatives such as niacin and nicotinamide reduce phosphate absorption, serum phosphate, and FGF23. This novel therapeutic approach will be tested in the CKD Optimal Management with Binders and Nicotinamide (COMBINE) Study, with intermediate cardiovascular disease endpoints to include left ventricular hypertrophy (LVH), vascular calcification, and CKD progression.
Obesity and Metabolic Syndrome Obesity and associated metabolic syndrome, the results of Western dietary habits and sedentary lifestyles, exist at epidemic proportions in the US and are spreading worldwide. 222 The continuous increase in obesity decreases life expectancy and general health. 223 Obese participants are at greater risks for hypertension, insulin resistance and diabetes, hyperlipidemia, various cancers, and coronary vascular disease. 224 Obesity and metabolic syndrome are also associated with pathologic renal changes and decreased renal function. 224,225
Recently, the likely causes of the obesity epidemic were reviewed.226 Attempts to battle the problem have concentrated on decreasing fast food and trans fats intakes by providing nutritional information in stores and restaurants as well as reducing the consumption of soft drinks and high-fructose corn syrup.222 Numerous studies have associated increased trans fats intake with an increased risk of coronary disease.227–229 The intake of high-fructose corn syrup causes lipid abnormalities and hepatic insulin resistance.230 Epidemiologic studies show that the consumption of beverages containing a combination of sugars (including fructose) are associated with increases in body weight, metabolic syndrome, and cardiovascular disease.231 Similarly, the heightened use of artificial sweeteners is associated with obesity.232 Increasing evidence suggests that artificial sweeteners do not activate food reward pathways in the same manner as natural sweeteners,233 as demonstrated by the lack of the prolonged signal depression in the hypothalamus observed following glucose ingestion. Finally, sucrose ingestion, compared with saccharin ingestion, results in the greater activation of the higher gustatory areas such as the insula, orbitofrontal cortex, and the amygdala; this information might be useful for limiting energy intake.233–235
Obesity. The incidence of obesity, defined as a BMI of ≥30 kg/m2, has doubled since 1960. This condition affects one-third of the adult population in the US. The rise in overweight children from 6% to 19% over the past 25 years is even more alarming.236 Obesity is rapidly exceeding smoking as the leading cause of preventable death in the US.224 Eight studies have related excess body weight to the development of CKD and ESRD.237 An analysis of 320,252 members of the Kaiser Permanente Health System demonstrated that obesity is a risk factor for ESRD, with adjusted relative risks of 1.87, 3.57, 6.12, and 7.07 for those with BMIs of 25–29.9, 30–34.9, 35–39.9, and >40 kg/m2, respectively.238 Although the World Health Organization continues to use BMI to define obesity, the waist-to-hip ratio has been shown to predict more accurately the myocardial infarct risk worldwide.239 The link between obesity (defined by waist-to-hip ratio) and CKD has been reported.240 Even lean individuals with a high waist-to-hip ratio were at risk for developing microalbuminuria and a reduced estimated GFR.241 Obesity-related focal segmental glomerulosclerosis has also been described.242 The pathogenesis of this disorder is likely related to hyperfiltration, with increases in kidney mass and a glomerular hypertrophy effect. Hyperfiltration and increased filtration fraction are surrogate markers for elevated glomerular capillary pressures, which eventually result in obesity-associated glomerulosclerosis.243 Obesity-associated focal segmental glomerulosclerosis is associated with a lower rate of nephrotic syndrome and a more indolent course than idiopathic focal segmental glomerulosclerosis.242
Paradoxically, a strong association between increased body mass index (BMI) and lower mortality has been described in numerous studies of patients with stage 5 CKD undergoing maintenance hemodialysis with the benefits of a larger size extending into morbid obesity (BMI>35 kg/m2).244 This has been extended by two studies of patients with CKD where low BMI predicted greater mortality, whereas increased BMI was associated with greater survival even after adjustment for known confounding variables.245,246 The reasons for this association have not been determined.
Metabolic Syndrome. Associations between metabolic syndrome and both CKD and microalbuminuria have also been found in numerous studies, and Peralta et al. recently reviewed these relationships.247 After examining the data of 6,217 adults in the National Health and Nutrition Examination Survey III (NHANES), 24.7% of whom had metabolic syndrome, Chen et al. demonstrated graded relationships between the components of metabolic syndrome and the risks for CKD and microalbuminuria.248 Due to the cross-sectional nature of the study, determination of the temporal relationship between metabolic syndrome and CKD was not possible.247 The Atherosclerosis Risk in Communities Study (ARIC) examined more than 15,000 individuals and found that 21% (n=2,110) and 7% (n=691) developed metabolic syndrome and CKD, respectively, over a 9-year period; moreover, a similar graded relationship was found between the components of the syndrome and the risk for CKD. The odds ratio for the rate of CKD among participants with metabolic syndrome was 1.24 (95% CI 1.01–1.51).
Similarly, experimental hyperlipidemia models have demonstrated associations among progressive kidney damage, atherosclerosis, focal segmental glomerulosclerosis, and tubule-interstitial disease.249 Interestingly, a recent investigation of 19,246 participants in the southern US documented an association between a high saturated fat intake and albuminuria; however, no relationship was found with regard to decreased GFR.250 Moreover, an increased fructose intake (≥74 g/day) was implicated in obesity, metabolic syndrome, uric acid elevations, and hypertension; furthermore, it was a risk factor for kidney disease.251 High-fructose diets in animals led to renal hypertrophy, tubular cell proliferation, and injury. In a remnant kidney model, rats fed diets high in fructose developed metabolic syndrome and kidney disease progression.252 In humans, 6-week diets containing 25% fructose caused insulin resistance, visceral obesity, and abnormalities in serum lipids consistent with metabolic syndrome.253 The NHANES revealed an association between the ingestion of sugar-sweetened drinks and elevated uric acid levels; hypertension was also observed.253 Similarly, the Nurses’ Health Study found that ≥2 daily servings of artificially sweetened soda was independently associated with a ≥30% decline in estimated GFR over 11 years.254
A recent histopathologic study compared samples from 12 patients with metabolic syndrome undergoing nephrectomy for renal cancer with those from 12 controls.255 Samples from the patients with metabolic syndrome showed greater tubular atrophy, interstitial fibrosis, and arteriosclerosis as well as global and segmental glomerulosclerosis. These prominent interstitial changes led Saito et al. to postulate that the proximal tubular cell and, specifically, the multiligand megalin and cubilin receptors play a prominent role in the pathogenesis of this disorder.217
Welsh et al. recently engineered two mouse models lacking glomerular podocyte insulin receptors.256 Within 5 weeks, the animals began to show albuminuria and a shortening of the foot processes under electron microscopy. At 8 weeks, albuminuria, increased creatinine levels, the foci of segmental sclerosis, a thickening of the basement membranes, histologic evidence of apoptosis, and histopathologic features of diabetic nephropathy were observed, demonstrating the importance of podocyte insulin sensitivity in kidney function.257
According to the KDIGO guidelines, many patients with CKD should be treated with statins to prevent cardiovascular disease.53
Diabetes Mellitus. The importance of tight glycemic control to prevent kidney disease-related outcomes was recently demonstrated by the Diabetes Control and Complications Trial/Epidemiology of Diabetes Interventions and Complications Study (DCCT/ EDIC).258 The DCCT examined 1,441 participants with type 1 diabetes mellitus (1982–1993) assigned to intensive (median HgA1C 7.2%) versus conventional (9.1%) treatment for 6.5 years. Subsequently, participants were followed for >18 years in the observational EDIC. The intensive treatment used three or more daily insulin injections or insulin pump therapy guided by self-monitored glucose. During the DCCT, the intensive treatment reduced the rate of microalbuminuria (albumin excretion rate (AER) >40 mg/24 h) by 39% and that of macroalbuminuria (AER >300 mg/24 h) by 54% (24%–74%). During EDIC years 1–8, participants previously assigned to the DCCT intensive treatment experienced lower rates of microalbuminuria and macroalbuminuria, with risk reductions of 59% (30%–73%) and 84% (67%–92%), respectively. The beneficial effects of intensive therapy became evident at the end of the follow-up assessment, with reduced risks of impaired GFR (<60 mL/min) and hypertension of 50% (18%–69%) and 20% (6%–21%), respectively. The risk for retinopathy and neuropathy was also reduced, but not the risk for cardiovascular events.
With regard to type 2 diabetes, the kidney disease outcomes of the United Kingdom Prospective Diabetes Study (UKPDS),259 Kumamoto,260 Action in Diabetes and Vascular Disease Trial (ADVANCE),261 and Action to Control Cardiovascular Risk in Diabetes (ACCORD)262 trials are consistent with those of the DCCT for patients with type 1 diabetes.258 These analyses account for the relative differences in hemoglobin A1C achieved between treatment groups and the differences in study duration. A meta-analysis of type 2 diabetics263 featured 28 trials that included 34,912 participants with type 2 diabetes who were randomly assigned to an intensive glycemic control group (n=18,717) or a conventional glycemic control group (n=16,195). Targeting intensive glycemic control reduced the risk of microvascular complications (i.e. nephropathy and retinopathy) but increased the risks for hypoglycemia and serious adverse events. Tight glucose control confers long-term benefits regarding the prevention of progressive diabetic kidney disease.
Many individuals are not candidates for intensive glucose control in view of frequent episodes of hypoglycemia, impaired cognitive status, multiple comorbidities, and shortened life expectancies. Clinical guidelines have therefore recommended hemoglobin A1C targets as follows: specifically “individualized” care with A1C ~6.5% for healthy, young patients; <7% in older individuals or those with comorbid conditions, and <8% in older individuals with just a few years of life expectancy.264,265
Other Risk Factors Studies recently have identified uric acid, 266–268 acidosis, 269–272 and acute kidney injury 273,274 as potentially modifiable risk factors for CKD. |
1. Cooney MT, Dudina A, D’Agostino R, Graham IM. Cardiovascular risk-estimation systems in primary prevention: do they differ? Do they make a difference? Can we see the future? Circulation. 2010;122:300–10. http://dx.doi.org/10.1161/CIRCULATIONAHA.109.852756. 2. National Institutes of Health, National Heart, Lung, and Blood Institute. Disease Statistics. Bethesda, MD: National Institutes of Health, National Heart, Lung, and Blood Institute; 2013 [Accessed June 23, 2015 ]. Fact Book Fiscal Year 2012. pp. 33–52. Available at: http://1.usa.gov/%201MPNZEf. 4. Kannel WB, Dawber TR, Kagan A, Revotskie N, Stokes J 3rd. Factors of risk in the development of coronary heart disease--six year follow-up experience. The Framingham Study. Ann Intern Med. 1961;55:33–50. http://dx.doi.org/10.7326/0003-4819-55-1-33. 8. Batsis JA, Lopez-Jimenez F. Cardiovascular risk assessment--from individual risk prediction to estimation of global risk and change in risk in the population. BMC Med. 2010;8:29. http://dx.doi.org/10.1186/1741-7015-8-29. 9. Humphries SE, Drenos F, Ken-Dror G, Talmud PJ. Coronary heart disease risk prediction in the era of genome-wide association studies: current status and what the future holds. Circulation. 2010;121:2235–48. http://dx.doi.org/10.1161/CIRCULATIONAHA.109.914192. 13. Alexander S. Medical miracle and a moral burden of a small committee: they decide who lives, who dies. Life. 1962:102–25. 16. United States Renal Data System, 2014 Annual Data Report: Epidemiology of Kidney Disease in the United States. Bethesda, MD: National Institutes of Health, National Institute of Diabetes and Digestive and Kidney Diseases; 2014 [Accessed May 19, 2015 ]. Available at: http://www.usrds.org/adr.aspx. 19. Cain CD, Schroeder FC, Shankel SW, Mitchnick M, Schmertzler M, Bricker NS. Identification of xanthurenic acid 8-O-beta-D-glucoside and xanthurenic acid 8-O-sulfate as human natriuretic hormones. Proc Natl Acad Sci U S A. 2007;104:17873–8. http://dx.doi.org/10.1073/pnas.0705553104. 20. Hostetter TH, Olson JL, Rennke HG, Venkatachalam MA, Brenner BM. Hyperfiltration in remnant nephrons: a potentially adverse response to renal ablation. Am J Physiol. 1981;241:F85–93. 21. Matsusaka T, Hymes J, Ichikawa I. Angiotensin in progressive renal diseases: theory and practice. J Am Soc Nephrol. 1996;7:2025–43. 22. Zatz R, Dunn BR, Meyer TW, Anderson S, Rennke HG, Brenner BM. Prevention of diabetic glomerulopathy by pharmacological amelioration of glomerular capillary hypertension. J Clin Invest. 1986;77:1925–30. http://dx.doi.org/10.1172/JCI112521. 24. Yoshida Y, Fogo A, Ichikawa I. Glomerular hemodynamic changes vs. hypertrophy in experimental glomerular sclerosis. Kidney Int. 1989;35:654–60. http://dx.doi.org/10.1038/ki.1989.35. 25. Levey AS, de Jong PE, Coresh J, et al. The definition, classification, and prognosis of chronic kidney disease: a KDIGO Controversies Conference report. Kidney Int. 2011;80:17–28. http://dx.doi.org/10.1038/ki.2010.483. 32. Lewis EJ, Hunsicker LG, Clarke WR, et al. Renoprotective effect of the angiotensin-receptor antagonist irbesartan in patients with nephropathy due to type 2 diabetes. N Engl J Med. 2001;345:851–60. http://dx.doi.org/10.1056/NEJMoa011303. 33. Brenner BM, Cooper ME, de Zeeuw D, et al. Effects of losartan on renal and cardiovascular outcomes in patients with type 2 diabetes and nephropathy. N Engl J Med. 2001;345:861–9. http://dx.doi.org/10.1056/NEJMoa011161. 35. Yusuf S, Sleight P, Pogue J, Bosch J, Davies R, Dagenais G. Effects of an angiotensin-converting-enzyme inhibitor, ramipril, on cardiovascular events in high-risk patients. The Heart Outcomes Prevention Evaluation Study Investigators. N Engl J Med. 2000;342:145–53. http://dx.doi.org/10.1056/NEJM200001203420301. 36. Ibsen H, Olsen MH, Wachtell K, et al. Reduction in albuminuria translates to reduction in cardiovascular events in hypertensive patients: losartan intervention for endpoint reduction in hypertension study. Hypertension. 2005;45:198–202. http://dx.doi.org/10.1161/01.HYP.0000154082.72286.2a. 37. Chamnan P, Simmons RK, Khaw KT, Wareham NJ, Griffin SJ. Estimating the population impact of screening strategies for identifying and treating people at high risk of cardiovascular disease: modelling study. BMJ. 2010;340:c1693. 41. Weiner DE, Tighiouart H, Amin MG, et al. Chronic kidney disease as a risk factor for cardiovascular disease and all-cause mortality: a pooled analysis of community-based studies. J Am Soc Nephrol. 2004;15:1307–15. http://dx.doi.org/10.1097/01.ASN.0000123691.46138.E2. 43. Go AS, Chertow GM, Fan D, McCulloch CE, Hsu CY. Chronic kidney disease and the risks of death, cardiovascular events, and hospitalization. N Engl J Med. 2004;351:1296–305. http://dx.doi.org/10.1056/NEJMoa041031. 44. Matsushita K, van der Velde M, Astor BC, et al. Association of estimated glomerular filtration rate and albuminuria with all-cause and cardiovascular mortality in general population cohorts: a collaborative meta-analysis. Lancet. 2010;375:2073–81. http://dx.doi.org/10.1016/S0140-6736(10)60674-5. 46. DeFilippis AP, Kramer HJ, Katz R, et al. Association between coronary artery calcification progression and microalbuminuria: the MESA study. JACC Cardiovasc Imaging. 2010;3:595–604. http://dx.doi.org/10.1016/j.jcmg.2010.01.008. 47. Hallan S, Astor B, Romundstad S, Aasarod K, Kvenild K, Coresh J. Association of kidney function and albuminuria with cardiovascular mortality in older vs younger individuals: The HUNT II Study. Arch Intern Med. 2007;167:2490–6. http://dx.doi.org/10.1001/archinte.167.22.2490. 48. Foley RN, Wang C, Snyder JJ, Rule AD, Collins AJ. Kidney function and risk triage in adults: threshold values and hierarchical importance. Kidney Int. 2010;79:99–111. http://dx.doi.org/10.1038/ki.2010.291. 50. Van Biesen W, De Bacquer D, Verbeke F, Delanghe J, Lameire N, Vanholder R. The glomerular filtration rate in an apparently healthy population and its relation with cardiovascular mortality during 10 years. Eur Heart J. 2007;28:478–83. http://dx.doi.org/10.1093/eurheartj/ehl455. 53. Wanner C, Tonelli M. Kidney Disease: Improving Global Outcomes Lipid Guideline Development Work Group Members. KDIGO Clinical Practice Guideline for Lipid Management in Chronic Kidney Disease. Kidney Int. 2014;85:1303–9. http://dx.doi.org/10.1038/ki.2014.31. 57. Ruggenenti P, Perna A, Gherardi G, Benini R, Remuzzi G. Chronic proteinuric nephropathies: outcomes and response to treatment in a prospective cohort of 352 patients with different patterns of renal injury. Am J Kidney Dis. 2000;35:1155–65. http://dx.doi.org/10.1016/S0272-6386(00)70054-0. 58. Schrier RW, Estacio RO, Esler A, Mehler P. Effects of aggressive blood pressure control in normotensive type 2 diabetic patients on albuminuria, retinopathy and strokes. Kidney Int. 2002;61:1086–97. http://dx.doi.org/10.1046/j.1523-1755.2002.00213.x. 59. Wright JT Jr, Bakris G, Greene T, et al. Effect of blood pressure lowering and antihypertensive drug class on progression of hypertensive kidney disease: results from the AASK trial. JAMA. 2002;288:2421–31. http://dx.doi.org/10.1001/jama.288.19.2421. 61. Jafar TH, Stark PC, Schmid CH, et al. Progression of chronic kidney disease: the role of blood pressure control, proteinuria, and angiotensin-converting enzyme inhibition: a patient-level meta-analysis. Ann Intern Med. 2003;139:244–52. http://dx.doi.org/10.7326/0003-4819-139-4-200308190-00006. 63. Lewis EJ, Hunsicker LG, Bain RP, Rohde RD. The effect of angiotensin-converting-enzyme inhibition on diabetic nephropathy. The Collaborative Study Group. N Engl J Med. 1993;329:1456–62. http://dx.doi.org/10.1056/NEJM199311113292004. 64. Hoy WE, Wang Z, VanBuynder P, Baker PR, Mathews JD. The natural history of renal disease in Australian Aborigines. Part 1. Changes in albuminuria and glomerular filtration rate over time. Kidney Int. 2001;60:243–8. http://dx.doi.org/10.1046/j.1523-1755.2001.00792.x. 70. Nakao N, Yoshimura A, Morita H, Takada M, Kayano T, Ideura T. Combination treatment of angiotensin-II receptor blocker and angiotensin-converting-enzyme inhibitor in non-diabetic renal disease (COOPERATE): a randomised controlled trial. Lancet. 2003;361:117–24. http://dx.doi.org/10.1016/S0140-6736(03)12229-5. 72. Parving HH, Lehnert H, Brochner-Mortensen J, Gomis R, Andersen S, Arner P. The effect of irbesartan on the development of diabetic nephropathy in patients with type 2 diabetes. N Engl J Med. 2001;345:870–8. http://dx.doi.org/10.1056/NEJMoa011489. 73. Barnett AH, Bain SC, Bouter P, et al. Angiotensin-receptor blockade versus converting-enzyme inhibition in type 2 diabetes and nephropathy. N Engl J Med. 2004;351:1952–61. http://dx.doi.org/10.1056/NEJMoa042274. 75. Eijkelkamp WB, Zhang Z, Remuzzi G, et al. Albuminuria is a target for renoprotective therapy independent from blood pressure in patients with type 2 diabetic nephropathy: post hoc analysis from the Reduction of Endpoints in NIDDM with the Angiotensin II Antagonist Losartan (RENAAL) trial. J Am Soc Nephrol. 2007;18:1540–6. http://dx.doi.org/10.1681/ASN.2006050445. 77. Teo K, Yusuf S, Sleight P, et al. Rationale, design, and baseline characteristics of 2 large, simple, randomized trials evaluating telmisartan, ramipril, and their combination in high-risk patients: the Ongoing Telmisartan Alone and in Combination with Ramipril Global Endpoint Trial/Telmisartan Randomized Assessment Study in ACE Intolerant Subjects with Cardiovascular Disease (ONTARGET/TRANSCEND) trials. Am Heart J. 2004;148:52–61. http://dx.doi.org/10.1016/j.ahj.2004.03.020. 79. Ontarget Investigators. Yusuf S, Teo KK, et al. Telmisartan, ramipril, or both in patients at high risk for vascular events. N Engl J Med. 2008;358:1547–59. http://dx.doi.org/10.1056/NEJMoa0801317. 83. Hou FF, Xie D, Zhang X, et al. Renoprotection of Optimal Antiproteinuric Doses (ROAD) Study: a randomized controlled study of benazepril and losar tan in chronic renal insufficiency. J Am Soc Nephrol. 2007;18:1889–98. http://dx.doi.org/10.1681/ASN.2006121372. 84. Rossing K, Schjoedt KJ, Jensen BR, Boomsma F, Parving HH. Enhanced renoprotective effects of ultrahigh doses of irbesartan in patients with type 2 diabetes and microalbuminuria. Kidney Int. 2005;68:1190–8. http://dx.doi.org/10.1111/j.1523-1755.2005.00511.x. 85. Weir MR, Hollenberg NK, Zappe DH, et al. Antihypertensive effects of double the maximum dose of valsartan in African-American patients with type 2 diabetes mellitus and albuminuria. J Hypertens. 2010;28:186–93. http://dx.doi.org/10.1097/HJH.0b013e328332bd61. 86. Schmieder RE, Klingbeil AU, Fleischmann EH, Veelken R, Delles C. Additional antiproteinuric effect of ultrahigh dose candesartan: a double-blind, randomized, prospective study. J Am Soc Nephrol. 2005;16:3038–45. http://dx.doi.org/10.1681/ASN.2005020138. 88. Persson F, Rossing P, Reinhard H, et al. Optimal antiproteinuric dose of aliskiren in type 2 diabetes mellitus: a randomised crossover trial. Diabetologia. 2010;53:1576–80. http://dx.doi.org/10.1007/s00125-010-1789-6. 89. Schjoedt KJ, Astrup AS, Persson F, et al. Optimal dose of lisinopril for renoprotection in type 1 diabetic patients with diabetic nephropathy: a randomised crossover trial. Diabetologia. 2009;52:46–9. http://dx.doi.org/10.1007/s00125-008-1184-8. 90. Andersen S, Rossing P, Juhl TR, Deinum J, Parving HH. Optimal dose of losartan for renoprotection in diabetic nephropathy. Nephrol Dial Transplant. 2002;17:1413–18. http://dx.doi.org/10.1093/ndt/17.8.1413. 92. Buter H, Hemmelder MH, Navis G, de Jong PE, de Zeeuw D. The blunting of the antiproteinuric efficacy of ACE inhibition by high sodium intake can be restored by hydrochlorothiazide. Nephrol Dial Transplant. 1998;13:1682–5. http://dx.doi.org/10.1093/ndt/13.7.1682. 93. Esnault VL, Ekhlas A, Delcroix C, Moutel MG, Nguyen JM. Diuretic and enhanced sodium restriction results in improved antiproteinuric response to RAS blocking agents. J Am Soc Nephrol. 2005;16:474–81. http://dx.doi.org/10.1681/ASN.2004060505. 94. Navis G, de Jong PE, Donker AJ, van der Hem GK, de Zeeuw D. Moderate sodium restriction in hypertensive subjects: renal effects of ACE-inhibition. Kidney Int. 1987;31:815–19. http://dx.doi.org/10.1038/ki.1987.71. 96. Vogt L, Waanders F, Boomsma F, de Zeeuw D, Navis G. Effects of dietary sodium and hydrochlorothiazide on the antiproteinuric efficacy of losartan. J Am Soc Nephrol. 2008;19:999–1007. http://dx.doi.org/10.1681/ASN.2007060693. 97. Weir MR, Bakris GL, Bushinsky DA, et al. Patiromer in patients with kidney disease and hyperkalemia receiving RAAS inhibitors. N Engl J Med. 2015;372:211–21. http://dx.doi.org/10.1056/NEJMoa1410853. 99. Bakris GL, Williams M, Dworkin L, et al. Preserving renal function in adults with hypertension and diabetes: a consensus approach. National Kidney Foundation Hypertension and Diabetes Executive Committees Working Group. Am J Kidney Dis. 2000;36:646–61. http://dx.doi.org/10.1053/ajkd.2000.16225. 101. Xie D, Hou FF, Fu BL, Zhang X, Liang M. High level of proteinuria during treatment with renin-angiotensin inhibitors is a strong predictor of renal out-come in nondiabetic kidney disease. J Clin Pharmacol. 2011;51:1025–34. http://dx.doi.org/10.1177/0091270010377502. 103. Effect of enalapril on survival in patients with reduced left ventricular ejection fractions and congestive heart failure. The SOLVD Investigators. N Engl J Med. 1991;325:293–302. http://dx.doi.org/10.1056/NEJM199108013250501. 104. Jong P, Yusuf S, Rousseau MF, Ahn SA, Bangdiwala SI. Effect of enalapril on 12-year survival and life expectancy in patients with left ventricular systolic dysfunction: a follow-up study. Lancet. 2003;361:1843–8. http://dx.doi.org/10.1016/S0140-6736(03)13501-5. 105. Dagenais GR, Pogue J, Fox K, Simoons ML, Yusuf S. Angiotensin-converting-enzyme inhibitors in stable vascular disease without left ventricular systolic dysfunction or heart failure: a combined analysis of three trials. Lancet. 2006;368:581–8. http://dx.doi.org/10.1016/S0140-6736(06)69201-5. 106. Effects of ramipril on cardiovascular and microvascular outcomes in people with diabetes mellitus: results of the HOPE study and MICRO-HOPE sub-study. Heart Outcomes Prevention Evaluation Study Investigators. Lancet. 2000;355:253–9. http://dx.doi.org/10.1016/S0140-6736(99)12323-7. 108. Rachmani R, Levi Z, Lidar M, Slavachevski I, Half-Onn E, Ravid M. Considerations about the threshold value of microalbuminuria in patients with diabetes mellitus: lessons from an 8-year follow-up study of 599 patients. Diabetes Res Clin Pract. 2000;49:187–94. http://dx.doi.org/10.1016/S0168-8227(00)00155-8. 109. Pereira M, Lunet N, Azevedo A, Barros H. Differences in prevalence, awareness, treatment and control of hypertension between developing and developed countries. J Hypertens. 2009;27:963–75. http://dx.doi.org/10.1097/HJH.0b013e3283282f65. 114. Walker WG, Neaton JD, Cutler JA, Neuwirth R, Cohen JD. Renal function change in hypertensive members of the Multiple Risk Factor Intervention Trial. Racial and treatment effects. The MRFIT Research Group. JAMA. 1992;268:3085–91. http://dx.doi.org/10.1001/jama.1992.03490210067037. 118. Berl T, Hunsicker LG, Lewis JB, et al. Impact of achieved blood pressure on cardiovascular outcomes in the Irbesartan Diabetic Nephropathy Trial. J Am Soc Nephrol. 2005;16:2170–9. http://dx.doi.org/10.1681/ASN.2004090763. 119. Cooper-DeHoff RM, Gong Y, Handberg EM, et al. Tight blood pressure control and cardiovascular outcomes among hypertensive patients with diabetes and coronary artery disease. JAMA. 2010;304:61–8. http://dx.doi.org/10.1001/jama.2010.884. 120. Mancia G. Effects of intensive blood pressure control in the management of patients with type 2 diabetes mellitus in the Action to Control Cardiovascular Risk in Diabetes (ACCORD) trial. Circulation. 2010;122:847–9. http://dx.doi.org/10.1161/CIRCULATIONAHA.110.960120. 121. Zanchetti A, Grassi G, Mancia G. When should anti-hypertensive drug treatment be initiated and to what levels should systolic blood pressure be lowered? A critical reappraisal. J Hypertens. 2009;27:923–34. http://dx.doi.org/10.1097/HJH.0b013e32832aa6b5. 122. Ruggenenti P, Perna A, Loriga G, et al. Blood-pressure control for renoprotection in patients with non-diabetic chronic renal disease (REIN-2): multicentre, randomised controlled trial. Lancet. 2005;365:939–46. http://dx.doi.org/10.1016/S0140-6736(05)71082-5. 123. Verdecchia P, Staessen JA, Angeli F, et al. Usual versus tight control of systolic blood pressure in non-diabetic patients with hypertension (Cardio-Sis): an open-label randomised trial. Lancet. 2009;374:525–33. http://dx.doi.org/10.1016/S0140-6736(09)61340-4. 125. Rahman M, Pressel S, Davis BR, et al. Renal outcomes in high-risk hypertensive patients treated with an angiotensin-converting enzyme inhibitor or a calcium channel blocker vs a diuretic: a report from the Antihypertensive and Lipid-Lowering Treatment to Prevent Heart Attack Trial (ALLHAT). Arch Intern Med. 2005;165:936–46. http://dx.doi.org/10.1001/archinte.165.8.936. 130. Gentile G, Remuzzi G, Ruggenenti P. Dual renin-angiotensin system blockade for nephroprotection: still under scrutiny. Nephron. 2015;129:39–41. http://dx.doi.org/10.1159/000368331. 132. Marchand GR. Effect of parathyroid hormone on the determinants of glomerular filtration in dogs. Am J Physiol. 1985;248:F482–6. 134. Kovesdy CP, Ahmadzadeh S, Anderson JE, Kalantar-Zadeh K. Secondary hyperparathyroidism is associated with higher mortality in men with moderate to severe chronic kidney disease. Kidney Int. 2008;73:1296–302. http://dx.doi.org/10.1038/ki.2008.64. 135. Schumock GT, Andress DL, Marx SE, Sterz R, Joyce AT, Kalantar-Zadeh K. Association of secondary hyperparathyroidism with CKD progression, health care costs and survival in diabetic predialysis CKD patients. Nephron Clin Pract. 2009;113:c54–61. http://dx.doi.org/10.1159/000228076. 136. Costa-Hong V, Jorgetti V, Gowdak LH, Moyses RM, Krieger EM, De Lima JJ. Parathyroidectomy reduces cardiovascular events and mortality in renal hyperparathyroidism. Surgery. 2007;142:699–703. http://dx.doi.org/10.1016/j.surg.2007.06.015. 138. Loghman-Adham M. Role of phosphate retention in the progression of renal failure. J Lab Clin Med. 1993;122:16–26. 140. Norris KC, Greene T, Kopple J, et al. Baseline predictors of renal disease progression in the African American Study of Hypertension and Kidney Disease. J Am Soc Nephrol. 2006;17:2928–36. http://dx.doi.org/10.1681/ASN.2005101101. 141. Schwarz S, Trivedi BK, Kalantar-Zadeh K, Kovesdy CP. Association of disorders in mineral metabolism with progression of chronic kidney disease. Clin J Am Soc Nephrol. 2006;1:825–31. http://dx.doi.org/10.2215/CJN.02101205. 142. Fliser D, Kollerits B, Neyer U, et al. Fibroblast growth factor 23 (FGF23) predicts progression of chronic kidney disease: the Mild to Moderate Kidney Disease (MMKD) Study. J Am Soc Nephrol. 2007;18:2600–8. http://dx.doi.org/10.1681/ASN.2006080936. 143. Voormolen N, Noordzij M, Grootendorst DC, et al. High plasma phosphate as a risk factor for decline in renal function and mortality in predialysis patients. Nephrol Dial Transplant. 2007;22:2909–16. http://dx.doi.org/10.1093/ndt/gfm286. 144. Levin A, Djurdjev O, Beaulieu M, Er L. Variability and risk factors for kidney disease progression and death following attainment of stage 4 CKD in a referred cohort. Am J Kidney Dis. 2008;52:661–71. http://dx.doi.org/10.1053/j.ajkd.2008.06.023. 145. Noori N, Sims JJ, Kopple JD, et al. Organic and inorganic dietary phosphorus and its management in chronic kidney disease. Iran J Kidney Dis. 2010;4:89–100. 148. Nagano N, Miyata S, Obana S, et al. Sevelamer hydrochloride, a phosphate binder, protects against deterioration of renal function in rats with progressive chronic renal insufficiency. Nephrol Dial Transplant. 2003;18:2014–23. http://dx.doi.org/10.1093/ndt/gfg309. 150. Kestenbaum B, Sampson JN, Rudser KD, et al. Serum phosphate levels and mortality risk among people with chronic kidney disease. J Am Soc Nephrol. 2005;16:520–8. http://dx.doi.org/10.1681/ASN.2004070602. 151. Stavroulopoulos A, Porter CJ, Pointon K, Monaghan JM, Roe SD, Cassidy MJ. Evolution of coronary artery calcification in patients with chronic kidney disease Stages 3 and 4, with and without diabetes. Nephrol Dial Transplant. 2011;26:2582–9. http://dx.doi.org/10.1093/ndt/gfq751. 152. Ix JH, De Boer IH, Peralta CA, et al. Serum phosphorus concentrations and arterial stiffness among individuals with normal kidney function to moderate kidney disease in MESA. Clin J Am Soc Nephrol. 2009;4:609–15. http://dx.doi.org/10.2215/CJN.04100808. 154. de Boer IH, Rue TC, Kestenbaum B. Serum phosphorus concentrations in the third National Health and Nutrition Examination Survey (NHANES III). Am J Kidney Dis. 2009;53:399–407. http://dx.doi.org/10.1053/j.ajkd.2008.07.036. 155. Dhingra R, Sullivan LM, Fox CS, et al. Relations of serum phosphorus and calcium levels to the incidence of cardiovascular disease in the community. Arch Intern Med. 2007;167:879–85. http://dx.doi.org/10.1001/archinte.167.9.879. 156. Foley RN, Collins AJ, Ishani A, Kalra PA. Calcium-phosphate levels and cardiovascular disease in community-dwelling adults: the Atherosclerosis Risk in Communities (ARIC) Study. Am Heart J. 2008;156:556–63. http://dx.doi.org/10.1016/j.ahj.2008.05.016. 157. Foley RN, Collins AJ, Herzog CA, Ishani A, Kalra PA. Serum phosphorus levels associate with coronary atherosclerosis in young adults. J Am Soc Nephrol. 2009;20:397–404. http://dx.doi.org/10.1681/ASN.2008020141. 158. Foley RN, Collins AJ, Herzog CA, Ishani A, Kalra PA. Serum phosphate and left ventricular hypertrophy in young adults: the coronary artery risk development in young adults study. Kidney Blood Press Res. 2009;32:37–44. http://dx.doi.org/10.1159/000203348. 161. Dawson-Hughes B. Racial/ethnic considerations in making recommendations for vitamin D for adult and elderly men and women. Am J Clin Nutr. 2004;80:1763S–6S. 162. Nesby-O’Dell S, Scanlon KS, Cogswell ME, et al. Hypovitaminosis D prevalence and determinants among African American and white women of reproductive age: third National Health and Nutrition Examination Survey, 1988–1994. Am J Clin Nutr. 2002;76:187–92. 163. Bell NH, Epstein S, Greene A, Shary J, Oexmann MJ, Shaw S. Evidence for alteration of the vitamin D-endocrine system in obese subjects. J Clin Invest. 1985;76:370–3. http://dx.doi.org/10.1172/JCI111971. 165. Gupta A, Kallenbach LR, Zasuwa G, Divine GW. Race is a major determinant of secondary hyperparathyroidism in uremic patients. J Am Soc Nephrol. 2000;11:330–4. 167. Gutierrez OM, Isakova T, Andress DL, Levin A, Wolf M. Prevalence and severity of disordered mineral metabolism in Blacks with chronic kidney disease. Kidney Int. 2008;73:956–62. http://dx.doi.org/10.1038/ki.2008.4. 168. Gutierrez OM, Isakova T, Smith K, Epstein M, Patel N, Wolf M. Racial differences in postprandial mineral ion handling in health and in chronic kidney disease. Nephrol Dial Transplant. 2010;25:3970–7. http://dx.doi.org/10.1093/ndt/gfq316. 171. Beck L, Karaplis AC, Amizuka N, Hewson AS, Ozawa H, Tenenhouse HS. Targeted inactivation of Npt2 in mice leads to severe renal phosphate wasting, hyper-calciuria, and skeletal abnormalities. Proc Natl Acad Sci U S A. 1998;95:5372–7. http://dx.doi.org/10.1073/pnas.95.9.5372. 174. Schlingmann KP, Ruminska J, Kaufmann M, et al. Autosomal-recessive mutations in SLC34A1 encoding sodium-phosphate cotransporter 2A cause idiopathic infantile hypercalcemia. J Am Soc Nephrol. 2015 Jun 5; [Epub ahead of print] http://dx.doi.org/10.1681/asn.2014101025. 175. Rajagopal A, Braslavsky D, Lu JT, et al. Exome sequencing identifies a novel homozygous mutation in the phosphate transporter SLC34A1 in hypophosphatemia and nephrocalcinosis. J Clin Endocrinol Metab. 2014;99:E2451–6. http://dx.doi.org/10.1210/jc.2014-1517. 176. Kottgen A, Pattaro C, Boger CA, et al. New loci associated with kidney function and chronic kidney disease. Nat Genet. 2010;42:376–84. http://dx.doi.org/10.1038/ng.568. 178. Kuhlmann A, Haas CS, Gross ML, et al. 1,25-Dihydroxyvitamin D3 decreases podocyte loss and podocyte hypertrophy in the subtotally nephrectomized rat. Am J Physiol Renal Physiol. 2004;286:F526–33. http://dx.doi.org/10.1152/ajprenal.00316.2003. 180. Hirata M, Makibayashi K, Katsumata K, et al. 22-Oxacalcitriol prevents progressive glomerulosclerosis without adversely affecting calcium and phosphorus metabolism in subtotally nephrectomized rats. Nephrol Dial Transplant. 2002;17:2132–7. http://dx.doi.org/10.1093/ndt/17.12.2132. 181. Piecha G, Kokeny G, Nakagawa K, et al. Calcimimetic R-568 or calcitriol: equally beneficial on progression of renal damage in subtotally nephrectomized rats. Am J Physiol Renal Physiol. 2008;294:F748–57. http://dx.doi.org/10.1152/ajprenal.00220.2007. 182. Coen G, Mazzaferro S, Manni M, et al. No acceleration and possibly slower progression of renal failure during calcitriol treatment in predialysis chronic renal failure. Nephrol Dial Transplant. 1994;9:1520. 186. Fishbane S, Chittineni H, Packman M, Dutka P, Ali N, Durie N. Oral paricalcitol in the treatment of patients with CKD and proteinuria: a randomized trial. Am J Kidney Dis. 2009;54:647–52. http://dx.doi.org/10.1053/j.ajkd.2009.04.036. 187. Tan X, Wen X, Liu Y. Paricalcitol inhibits renal inflammation by promoting vitamin D receptor-mediated sequestration of NF-kappaB signaling. J Am Soc Nephrol. 2008;19:1741–52. http://dx.doi.org/10.1681/ASN.2007060666. 188. Mizobuchi M, Morrissey J, Finch JL, et al. Combination therapy with an angiotensin-converting enzyme inhibitor and a vitamin D analog suppresses the progression of renal insufficiency in uremic rats. J Am Soc Nephrol. 2007;18:1796–806. http://dx.doi.org/10.1681/ASN.2006091028. 189. de Zeeuw D, Agarwal R, Amdahl M, et al. Selective vitamin D receptor activation with paricalcitol for reduction of albuminuria in patients with type 2 diabetes (VITAL study): a randomised controlled trial. Lancet. 2010;376:1543–51. http://dx.doi.org/10.1016/S0140-6736(10)61032-X. 190. Levin A, Bakris GL, Molitch M, et al. Prevalence of abnormal serum vitamin D, PTH, calcium, and phosphorus in patients with chronic kidney disease: results of the study to evaluate early kidney disease. Kidney Int. 2007;71:31–8. http://dx.doi.org/10.1038/sj.ki.5002009. 193. Teng M, Wolf M, Lowrie E, Ofsthun N, Lazarus JM, Thadhani R. Survival of patients undergoing hemodialysis with paricalcitol or calcitriol therapy. N Engl J Med. 2003;349:446–56. http://dx.doi.org/10.1056/NEJMoa022536. 195. Palmer SC, McGregor DO, Craig JC, Elder G, Macaskill P, Strippoli GF. Vitamin D compounds for people with chronic kidney disease not requiring dialysis. Cochrane Database Syst Rev. 2009;(4):CD008175. http://dx.doi.org/10.1002/14651858.CD008175. 196. Palmer SC, McGregor DO, Craig JC, Elder G, Macaskill P, Strippoli GF. Vitamin D compounds for people with chronic kidney disease requiring dialysis. Cochrane Database Syst Rev. 2009;(4):CD005633. http://dx.doi.org/10.1002/14651858.CD005633.pub2. 197. Al-Aly Z. Vitamin D as a novel nontraditional risk factor for mortality in hemodialysis patients: the need for randomized trials. Kidney Int. 2007;72:909–11. http://dx.doi.org/10.1038/sj.ki.5002544. 198. Gutierrez OM. Fibroblast growth factor 23 and disordered vitamin D metabolism in chronic kidney disease: updating the “trade-off” hypothesis. Clin J Am Soc Nephrol. 2010;5:1710–16. http://dx.doi.org/10.2215/CJN.02640310. 202. Gutierrez O, Isakova T, Rhee E, et al. Fibroblast growth factor-23 mitigates hyperphosphatemia but accentuates calcitriol deficiency in chronic kidney disease. J Am Soc Nephrol. 2005;16:2205–15. http://dx.doi.org/10.1681/ASN.2005010052. 203. Shigematsu T, Kazama JJ, Yamashita T, et al. Possible involvement of circulating fibroblast growth factor 23 in the development of secondary hyperparathyroidism associated with renal insufficiency. Am J Kidney Dis. 2004;44:250–6. http://dx.doi.org/10.1053/j.ajkd.2004.04.029. 204. Seiler S, Reichart B, Roth D, Seibert E, Fliser D, Heine GH. FGF-23 and future cardiovascular events in patients with chronic kidney disease before initiation of dialysis treatment. Nephrol Dial Transplant. 2010;25:3983–9. http://dx.doi.org/10.1093/ndt/gfq309. 205. Gutierrez OM, Mannstadt M, Isakova T, et al. Fibroblast growth factor 23 and mortality among patients undergoing hemodialysis. N Engl J Med. 2008;359:584–92. http://dx.doi.org/10.1056/NEJMoa0706130. 206. Jean G, Terrat JC, Vanel T, et al. High levels of serum fibroblast growth factor (FGF)-23 are associated with increased mortality in long haemodialysis patients. Nephrol Dial Transplant. 2009;24:2792–6. http://dx.doi.org/10.1093/ndt/gfp191. 207. Nasrallah MM, El-Shehaby AR, Salem MM, Osman NA, El Sheikh E, Sharaf El, Din UA. Fibroblast growth factor-23 (FGF-23) is independently correlated to aortic calcification in haemodialysis patients. Nephrol Dial Transplant. 2010;25:2679–85. http://dx.doi.org/10.1093/ndt/gfq089. 209. Wesseling-Perry K, Pereira RC, Wang H, et al. Relationship between plasma fibroblast growth factor-23 concentration and bone mineralization in children with renal failure on peritoneal dialysis. J Clin Endocrinol Metab. 2009;94:511–17. http://dx.doi.org/10.1210/jc.2008-0326. 211. Oliveira RB, Cancela AL, Graciolli FG, et al. Early control of PTH and FGF23 in normophosphatemic CKD patients: a new target in CKD-MBD therapy? Clin J Am Soc Nephrol. 2010;5:286–91. http://dx.doi.org/10.2215/CJN.05420709. 212. Muller D, Mehling H, Otto B, et al. Niacin lowers serum phosphate and increases HDL cholesterol in dialysis patients. Clin J Am Soc Nephrol. 2007;2:1249–54. http://dx.doi.org/10.2215/CJN.01470307. 213. Wetmore JB, Liu S, Krebill R, Menard R, Quarles LD. Effects of cinacalcet and concurrent low-dose vitamin D on FGF23 levels in ESRD. Clin J Am Soc Nephrol. 2010;5:110–16. http://dx.doi.org/10.2215/CJN.03630509. 214. Nishi H, Nii-Kono T, Nakanishi S, et al. Intravenous calcitriol therapy increases serum concentrations of fibroblast growth factor-23 in dialysis patients with secondary hyperparathyroidism. Nephron Clin Pract. 2005;101:c94–9. http://dx.doi.org/10.1159/000086347. 218. Mirza MA, Alsio J, Hammarstedt A, et al. Circulating fibroblast growth factor-23 is associated with fat mass and dyslipidemia in two independent cohorts of elderly individuals. Arterioscler Thromb Vasc Biol. 2011;31:219–27. http://dx.doi.org/10.1161/ATVBAHA.110.214619. 219. Frankenfield DL, Rocco MV, Roman SH, McClellan WM. Survival advantage for adult Hispanic hemodialysis patients? Findings from the end-stage renal disease clinical performance measures project. J Am Soc Nephrol. 2003;14:180–6. http://dx.doi.org/10.1097/01.ASN.0000037400.83593.E6. 221. Isakova T, Ix JH, Sprague SM, et al. Rationale and approaches to phosphate and fibroblast growth factor 23 reduction in CKD. J Am Soc Nephrol. 2015 May 12; pii: ASN2015020117. [Epub ahead of print]. 222. Hurt RT, Kulisek C, Buchanan LA, McClave SA. The obesity epidemic: challenges, health initiatives, and implications for gastroenterologists. Gastroenterol Hepatol (N Y). 2010;6:780–92. 223. Olshansky SJ, Passaro DJ, Hershow RC, et al. A potential decline in life expectancy in the United States in the 21st century. N Engl J Med. 2005;352:1138–45. http://dx.doi.org/10.1056/NEJMsr043743. 224. Guh DP, Zhang W, Bansback N, Amarsi Z, Birmingham CL, Anis AH. The incidence of comorbidities related to obesity and overweight: a systematic review and meta-analysis. BMC Public Health. 2009;9:88. http://dx.doi.org/10.1186/1471-2458-9-88. 228. Mente A, de Koning L, Shannon HS, Anand SS. A systematic review of the evidence supporting a causal link between dietary factors and coronary heart disease. Arch Intern Med. 2009;169:659–69. http://dx.doi.org/10.1001/archinternmed.2009.38. 230. Stanhope KL, Schwarz JM, Keim NL, et al. Consuming fructose-sweetened, not glucose-sweetened, beverages increases visceral adiposity and lipids and decreases insulin sensitivity in overweight/obese humans. J Clin Invest. 2009;119:1322–34. http://dx.doi.org/10.1172/JCI37385. 232. Yang Q. Gain weight by “going diet?” Artificial sweeteners and the neurobiology of sugar cravings: neuroscience 2010. Yale J Biol Med. 2010;83:101–8. 233. Smeets PA, de Graaf C, Stafleu A, van Osch MJ, van der Grond J. Functional magnetic resonance imaging of human hypothalamic responses to sweet taste and calories. Am J Clin Nutr. 2005;82:1011–16. 235. Cui M, Jiang P, Maillet E, Max M, Margolskee RF, Osman R. The heterodimeric sweet taste receptor has multiple potential ligand binding sites. Curr Pharm Des. 2006;12:4591–600. http://dx.doi.org/10.2174/138161206779010350. 241. Pinto-Sietsma SJ, Navis G, Janssen WM, de Zeeuw D, Gans RO, de Jong PE. A central body fat distribution is related to renal function impairment, even in lean subjects. Am J Kidney Dis. 2003;41:733–41. http://dx.doi.org/10.1016/S0272-6386(03)00020-9. 244. Kovesdy CP, Anderson JE. Reverse epidemiology in patients with chronic kidney disease who are not yet on dialysis. Semin Dial. 2007;20:566–9. 245. Evans M, Fryzek JP, Elinder CG, et al. The natural history of chronic renal failure: results from an unselected, population-based, inception cohort in Sweden. Am J Kidney Dis. 2005;46:863–70. http://dx.doi.org/10.1053/j.ajkd.2005.07.040. 246. Kovesdy CP, Anderson JE, Kalantar-Zadeh K. Paradoxical association between body mass index and mortality in men with CKD not yet on dialysis. Am J Kidney Dis. 2007;49:581–91. http://dx.doi.org/10.1053/j.ajkd.2007.02.277. 249. Joles JA, Kunter U, Janssen U, et al. Early mechanisms of renal injury in hypercholesterolemic or hypertriglyceridemic rats. J Am Soc Nephrol. 2000;11:669–83. 254. Lin J, Curhan GC. Associations of sugar and artificially sweetened soda with albuminuria and kidney function decline in women. Clin J Am Soc Nephrol. 2011;6:160–6. http://dx.doi.org/10.2215/CJN.03260410. 258. de Boer IH. DCCT EDIC Research Group. Kidney disease and related findings in the diabetes control and complications trial/epidemiology of diabetes interventions and complications study. Diabetes Care. 2014;37:24–30. http://dx.doi.org/10.2337/dc13-2113. 259. Intensive blood-glucose control with sulphonylureas or insulin compared with conventional treatment and risk of complications in patients with type 2 diabetes (UKPDS 33). UK Prospective Diabetes Study (UKPDS) Group. Lancet. 1998;352:837–53. http://dx.doi.org/10.1016/S0140-6736(98)07019-6. 260. Ohkubo Y, Kishikawa H, Araki E, et al. Intensive insulin therapy prevents the progression of diabetic microvascular complications in Japanese patients with non-insulin-dependent diabetes mellitus: a randomized prospective 6-year study. Diabetes Res Clin Pract. 1995;28:103–17. http://dx.doi.org/10.1016/0168-8227(95)01064-K. 262. Ismail-Beigi F, Craven T, Banerji MA, et al. Effect of intensive treatment of hyperglycaemia on microvascular outcomes in type 2 diabetes: an analysis of the ACCORD randomised trial. Lancet. 2010;376:419–30. http://dx.doi.org/10.1016/S0140-6736(10)60576-4. 263. Hemmingsen B, Lund SS, Gluud C, et al. Targeting intensive glycaemic control versus targeting conventional glycaemic control for type 2 diabetes mellitus. Cochrane Database Syst Rev. 2013;11:CD008143. 264. Inzucchi SE, Bergenstal RM, Buse JB, et al. Management of hyperglycemia in type 2 diabetes: a patient-centered approach: position statement of the American Diabetes Association (ADA) and the European Association for the Study of Diabetes (EASD). Diabetes Care. 2012;35:1364–79. http://dx.doi.org/10.2337/dc12-0413. 267. Ben-Dov IZ, Kark JD. Serum uric acid is a GFR-independent long-term predictor of acute and chronic renal insufficiency: the Jerusalem Lipid Research Clinic cohort study. Nephrol Dial Transplant. 2011;26:2558–66. http://dx.doi.org/10.1093/ndt/gfq740. 268. Kanji T, Gandhi M, Clase CM, Yang R. Urate lowering therapy to improve renal outcomes in patients with chronic kidney disease: systematic review and meta-analysis. BMC Nephrol. 2015;16:58. http://dx.doi.org/10.1186/s12882-015-0047-z. 272. Goldenstein L, Driver TH, Fried LF, et al. Serum bicarbonate concentrations and kidney disease progression in community-living elders: the Health, Aging, and Body Composition (Health ABC) Study. Am J Kidney Dis. 2014;64:542–9. http://dx.doi.org/10.1053/j.ajkd.2014.05.009. 273. Lo LJ, Go AS, Chertow GM, et al. Dialysis-requiring acute renal failure increases the risk of progressive chronic kidney disease. Kidney Int. 2009;76:893–9. http://dx.doi.org/10.1038/ki.2009.289. |