Vital Signs and COVID-19-Focused Sensors
The risk associated with disease spread to and through health care workers raised the need for telemetry of patients’ status across rooms, often without direct line of sight to some or all patients. The traditional non-invasive measurements of vital signs—blood pressure, heart rate, temperature, respiration rate, and oxygen saturation—had to be transferred to a remote control room. The lack of a sufficient amount of telemetry monitors that would allow a wired or wireless transfer of data to the control room is a key challenge, especially when considering the relatively sudden and unexpected deterioration patterns associated with the disease. This challenge is valid for both critical patients and patients with moderate-to-severe disease. The COVID-19 outbreak facilitated efforts to monitor vital signs without the need for physical contact as well as investigating old and new physiological measurements to allow appropriate patient monitoring.
As body temperature distribution and respiratory dynamics are among the primary indicators for COVID-19 infection,1,5–7 monitoring both these indicators without contact of the subject with a sensor from a distance of a few meters is a key challenge.8–10 Inferring core body temperature at a medical resolution (an error of about 0.3°C) from afar (more than 2 meters) is not a trivial task as it requires an accurate estimation of the core body temperature based on the detection of the absolute tissue temperature. Similarly, inferring remote and contactless respiratory dynamics is of paramount importance and a major challenge based on current technologies.
Thermal imaging is a method in which a camera’s sensor translates infrared signals into images, which represent the heat emitted from the captured objects.11 Uncooled bolometric sensors can sense radiation from objects at a temperature of −40°C to 140°C and can map body and tissue surface temperature remotely. Much research has been conducted in recent years in order to apply thermal imaging techniques for medical uses.11 The advantages of thermal imaging as a diagnostic tool arise from the fact that it is a low-cost, non-invasive, safe, and fast method that (most importantly in the COVID-19 case) does not require direct contact with the patient.
Besides fever detection,10,12 thermal imaging videos were used to infer physiological parameters related to respiratory patterns, such as breathing rate and tidal volume, by monitoring temperature changes around the nostrils.13–15 An additional use of thermal imaging that is currently under investigation is the assessment of peripheral perfusion. Changes in peripheral perfusion could be used for the detection of pathologies associated with damaged blood flow and for early diagnosis of shock states.11 Hand temperature distribution16 and foot thermal distribution were used to identify peripheral arterial disease.17,18 Differences between peripheral organs (ears) and core body temperature19 and thermal gradient along the arm20 were used for shock diagnosis.
One of the promising approaches to detect respiratory dynamics from a distance involves the usage of Doppler radar.21 These radars are not affected by factors such as temperature, light, and humidity but are extremely sensitive to motion and vibration in the vicinity of the subject. To overcome these limitations, a system that includes both Doppler radar and thermal imaging was developed.22 Signal integration from both systems was conducted by harnessing machine learning methods. The usage of machine learning occurs on two levels, technical and semantic. On the technical level, it is being used to improve the measurement of the physiological parameters. For example, deep convolutional networks are used for inferring the core temperature based on videos alone without any biophysical model inputs. On the semantic level, clinical status is inferred by aggregating the dynamical data (e.g. the entire body temperature distribution and the respiratory dynamics rather than just common parameters such as core temperature and respiratory rate). The system could be used at the hospital/emergency room entrance in order to test visitors or new patients at a distance without the need for personnel contact, serving as a first contact triage point. The application can allow to similarly trace vital signs in indoor environments (e.g. in emergency rooms). This system was co-developed during the COVID-19 crisis by the Israel Aerospace Industry (IAI) and the Technion and was applied at Rabin Medical Center emergency room, where it was used for remote patient monitoring. Another approach for remote vital sign monitoring was recently suggested by Binah.ai (Ramat-Gan, Israel), an approach relying solely on a smartphone camera either by taking a self-picture or by placing a finger over the camera for a few seconds.23
Another system utilizes a piezoelectric sensor integrated into a plate placed under the patient’s bed mattress (EarlySense, Ramat-Gan, Israel).24 The device senses the mechanical vibrations and movements created by the patient. Those are analyzed in order to provide contact-free, continuous monitoring of bed-ridden patients and may help in early detection of patient deteriorations.
It is prudent to wait for clinical validation of non-contact vital sign sensing of the above-mentioned systems.
Radar-based sensors (utilizing low-power radio frequency wavelength) are embedded in a system which is used for heart failure management by measuring lung fluid content (ReDS Heart Failure monitor™, Sensible Medical, Netanya, Israel).25–27 The system measures the dielectric properties of tissues. Low-power electromagnetic signals are emitted through the right mid-thorax, and the signals received after passing through the tissue reflect their combined dielectric properties. These, in turn, are mostly affected by the fluid content of the tissue in the path of the signal. The system is currently being investigated in COVID-19 patients as a tool that could potentially assist in following up the respiratory status of the COVID-19 patients and assist in managing those who require mechanical ventilation.
The high COVID-19 contamination risk, in addition to the shortage of protection gear and its encumbrance, led to a policy of limited entries into contaminated wards (usually one to two times during a 12-hour shift), in order to reduce staff–patient interactions unless an urgent need rises. This new condition in patient care required a new approach. When physicians and nurses contact the COVID-19 patients the heavy protection gear limits visibility and hearing. The use of stethoscope or direct visualization of the nasopharynx is discouraged, thus restricting physical exam to observation alone. The use of existing ultrasound systems (point-of-care ultrasound [POCUS], handheld) to assess patient pulmonary status and diagnose lung pathologies has been reported in COVID-19 patients.28,29 Audio and/or visually enhanced scopes (Tyto Care, Netanya, Israel) help clinicians evaluate patients’ cardiovascular, pulmonary, and upper respiratory tract status30 and enable a complete direct bedside physical exam. This represents an example of how a tool invented and marketed for remote patient home monitoring became relevant as a bedside tool.
Personnel Command and Control Systems
The expected overwhelming surge of patients during this crisis, the lack of staff trained in the medical intensive care unit (MICU), the requirement for operation within large halls (e.g. underground parking domains) using personal protective equipment (PPE), and the inherent uncertainties associated with a new pandemic may all coalesce to an austere environment with chaotic patterns of communication that may severely hamper the quality of care. Consequently, all entries to a quarantined domain have to be planned and executed flawlessly in order to avoid unnecessary re-entering, much like a spacewalk or entering a battle-zone. Similarly to these scenarios, there is a need for continuous and organized communication between the field teams at the ward or MICU and the control and command center (located in the non-infected environment) that need to integrate and operate the logistics and supportive para-clinical arms. In Israel, combat management strategies, communications, and other technologies were rapidly applied for the COVID-19 environments. Application of such technologies from the battle field to the medical field required mutual adaptations (of both the technology and medical teams) to maximize the operational capabilities of the team. The C
4I™ system is a command and control tactical system integrating computing, communication, and intelligence information (for health care applications this applies for patient sensors), developed for military use by Elbit Systems. A collaborative effort between Elbit and Rambam Health Care Campus modified the C
4I system for medical purposes and developed EX-TEAMS ™. EX-TEAMS is a dual WiFi and cellular and cloud-based communication and command platform. It was installed as a software app into push-to-talk mobile phones of all medical and para-clinical teams that operate in the hospital setting caring for COVID-19 patients. A key component of the system is the transformation of medical teams to predefined communication groups organized according to the clinical setting with strict hierarchical communication rules, thereby reducing the levels of the aforementioned chaos (
Figure 1). The cornerstone of the system is that each clinical team automatically communicate with the relevant team members using push-to-talk group communication. In addition, the system allows location tracking and automated location-based group switching, thereby allowing each member of the hospital clinical team arriving to a clinical domain to automatically join the relevant clinical team “micro-environment.” The location-based system allows hospital clinical command to better control key personnel (e.g. ICU physicians) and to allocate them according to their location and the clinical need. The addition of a location-based communication may also help to monitor the staff efforts in each clinical section and to allocate resources accordingly and help cut contamination trails if contamination occurs. The system prioritizes response efforts so as to provide efficient mission-focused allocation of workforce and assets, for example rapid assignment and deployment of emergency teams. Additionally, the system allows to receive real-time push-to-video, pictures, peer-to-peer calls, and chats across personnel and between the medical teams and the command and control units. Finally, a key challenge in the setting of COVID-19 is the limited ability to record clinical notes for the electronic medical record (EMR) under PPE. To overcome this challenge, the platform can potentially allow a voice medical record (VMR) to be recorded: a short, “tweet-like,” 1.5-minute voice clip, describing the clinical status of the patient, available to all personnel working in the facility. All the transmitted information is encrypted, maintaining medical confidentiality.
The implementation of the system in COVID-19 departments initially in Rambam and later on in 15 additional hospitals across Israel allowed clinical teams to easily communicate using PPE and to manage, control, and execute the complex tasks performed by a large and diverse workforce operating in a wide range of locations.31
Once each ward was equipped with a control room, then (as in usual times) all information available on the patients (directly and indirectly) can be entered into e-records. Connecting all control rooms to a central one at the hospitals and from each hospital to the national level was the next logical step in order to provide a reliable central real-time dashboard.
Central Command and Control System
During the early phases of the COVID-19 crisis, a real-time hospital command and control tool was evidently needed in order to manage the medical and logistical aspect of the crisis. To maximize hospitals’ efficiency a system integrating patients’ clinical data, medical staff status, critical clinical resources, and asset allocation into a single dashboard was required. The CoView™ System (a collaboration between the Department of Government Medical Centers within the Ministry of Health, the Directorate of Defense Research and Development within the Ministry of Defense, Israel defense forces, and specialists from the hi-tech industry) was developed for this goal, integrating defense concepts, big data analytics, and health care protocols.
The system reflects the status of all COVID-19 patients at all hospitals and admission facilities, in order to enable decision makers to make an efficient, evidence-based, optimized response. Using artificial intelligence algorithms, the system can analyze aggregated data from patient monitors and electronic charts, alerting medical staff to potential deterioration of specific patients, or analyze treatment protocols in specific hospitals (Figure 2). One could centrally control and optimize urgently needed (sometimes scarce or costly) resources such as PPE, drugs, mechanical ventilators, or extracorporeal membrane oxygenation (ECMO) systems. Moreover, professional advisors such as high-level experts can monitor the situation at each hospital, the treatment protocol used, and its efficacy and can help direct hospital staff across the country as needed.32,33
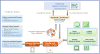 | Figure 2 Data Flow Scheme between Teams and Across the Different Operative and Command Levels |