| |
|
RMMJ
Rambam Maimonides Medical Journal
Rambam Health Care Campus 2017 October; 8(4): e0037.
ISSN: 2076-9172 Published online 2017 October 16. doi: 10.5041/RMMJ.10312 Special Issue on Novel Technologies in Urologic Investigation and Treatment Guest Editor: Gilad Amiel, M.D.
Multimodality Image Fusion with PSMA PET/CT and High-Intensity Focused Ultrasound Focal Therapy for Primary Diagnosis and Management of Prostate Cancer: A Planned Research Initiative Marcia C. Javitt, M.D.,1 Alexander Kravtsov, M.D.,2 Zohar Keidar, M.D., Ph.D.,3 Sobhi Abadi, M.D.,1 and Gilad Amiel, M.D.2 1Department of Medical Imaging, Rambam Health Care Campus, Haifa, Israel 2Department of Urology, Rambam Health Care Campus, Haifa, Israel 3Department of Nuclear Medicine, Rambam Health Care Campus, Haifa, Israel
|
Recent developments in diagnostic imaging herald a new approach to diagnosis and management of prostate cancer. Multimodality fusion that combines anatomic with functional imaging data has surpassed either of the two alone. This opens up the possibility to “find and fix” malignancy with greater accuracy than ever before. This is particularly important for prostate cancer because it is the most common male cancer in most developed countries. This article describes technical advances under investigation at our institution and others using multimodality image fusion of magnetic resonance imaging (MRI), transrectal ultrasound (TRUS), and PSMA PET/CT (defined as the combination of prostate-specific membrane antigen [PSMA], positron emission tomography [PET], and computed tomography [CT]) for personalized medicine in the diagnosis and focal therapy of prostate cancer with high-intensity focused ultrasound (HiFUS).
Keywords: Fusion, high-intensity focused ultrasound focal therapy, MRI, PSMA PET/CT, prostate cancer, TRUS |
In the United States, prostate cancer is the third most common cause of cancer death, with 161,360 new cases and 26,730 deaths expected in 2017.1 Worldwide 1,111,700 cases and 307,500 deaths are attributed to prostate cancer.2 While the incidence of prostate cancer spiked from about 1980 to 2000 due to prostate-specific antigen (PSA) blood test screening, recently the rates have declined dramatically because screening with PSA is no longer recommended for men of average risk.3 This is largely due to concerns about overdiagnosis and significant morbidity associated with overtreatment of prostate cancer. Overdiagnosis and overtreatment are estimated to occur in up to 42% of cases.3,4 There are also concerns about underdiagnosis of clinically significant high-risk cancers.3,4 In other words, currently there are no biomarkers that enable cost-effective screening for prostate cancer in average-risk patients.
At present, transrectal ultrasound (TRUS) with saturation biopsies is the standard of care for detection of prostate cancer, but it is limited due to sampling errors, overdetection of clinically insignificant cancers, and underdetection of some aggressive lesions. Multiparametric magnetic resonance imaging (mpMRI) combines anatomic and functional data, is routinely used for lesion detection and characterization, and enables risk stratification. However, MRI interpretation is labor-intensive, requiring significant expertise. Because positron emission tomography (PET) takes advantage of cell metabolism, cell division, and receptor binding to depict functional processes with well-known high sensitivity, new receptor-targeted agents for imaging prostate cancer (such as prostate-specific membrane antigen, PSMA) can add high specificity.
How can we take advantage of the complementary information provided by these different imaging modalities? Combining them for a single unified interpretation is now feasible using image fusion and hybrid techniques. This report will focus on the fusion of ultrasound (US), MRI, and PSMA PET/CT to detect clinically significant organ-confined prostate cancers, biopsy these target lesions, and institute focal therapy. The situation is analogous to the adoption of lumpectomy and radiation as breast-conserving surgery for breast cancer that occurred in the late 1980s in place of radical mastectomy in women.5 By sparing normal tissue, this “male lumpectomy” (i.e. focal therapy) reduces the risk of complications and accelerates recovery. One such focal therapy is high-intensity focused ultrasound (HiFUS).
This article describes the mandate for our institution’s interdepartmental research plan. We plan to employ multimodality image fusion for precise diagnosis, local staging, and focal therapy of organ-confined prostate cancer using HiFUS. Looking further forward, future multi-center randomized controlled trials comparing focal therapy to standard treatment with radical prostatectomy are needed to document equivalence or superiority of focal therapy before its widespread adoption can be recommended. |
Ultrasound Transrectal US ( Figure 1) with systematic biopsies combined with targeting of any focal hypoechoic foci is the standard of care for diagnosis of prostate cancer. Transrectal US is performed in real time for guidance of needle position and throw using needle tracking ( Figure 2). Transrectal US has no ionizing radiation, wide availability, but it is limited by operator dependence, motion, large body habitus, obscuration by gas, 6,7 and oversampling of the posterior gland with undersampling of the apex and anterior gland. 6–9 The more than 50% false positive biopsy results observed are usually from prostatitis and benign prostatic hypertrophy. 10 In addition, there is a significant false negative rate of at least 30% for clinically significant cancers (defined as Gleason score >7 and volume >0.5 cm 3). 11–13 Overdiagnosis of clinically insignificant lesions (defined as small cancers with Gleason scores <7) is also problematic. 4,14
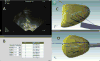 | Figure 2 3D TRUS-Guided Prostate Biopsy in a 47-Year-Old Male Uses 3D Prostate Model |
Multiparametric MRI By virtue of its anatomic and functional information, multiparametric MRI (mpMRI) (i.e. multiple pulse sequence MRI) offers significant advantages over TRUS for lesion detection, characterization, and local staging of prostate cancer. Use of endorectal coils and high-field (i.e. ≥3.0 Tesla) MR equipment is usually preferred due to increased signal-to-noise ratio and image quality.
Anatomic MRI pulse sequences are typically T1- and T2-weighted. The T2-weighted images are relatively high-resolution, showing zonal anatomy of the prostate to advantage. Functional sequences are diffusion-weighted (DWI) and dynamic contrast-enhanced (DCE) imaging. Apparent-diffusion coefficient (ADC) maps are derivatives of DWI measurements. Dynamic contrast-enhanced imaging is dynamic pre- and post-contrast-enhanced gradient echo imaging of the prostate. Most malignant lesions show focal early enhancement due to neovascularity, but this is not specific. Dynamic contrast-enhanced imaging is most useful when T2 and DWI images are indeterminate.
The multiple variables and pulse sequences in MRI are challenging to analyze and synthesize, resulting in a high interobserver variability.15,16 Fortunately the recently updated Prostate Imaging Reporting and Data System Version 2 (PI-RADS 2.0) is a guide to the performance, interpretation, and reporting of prostate MRI with risk stratification.17 This document will soon be accompanied by an atlas (PI-RADS v2 Atlas) that will likely be very useful to assist radiologists in pattern recognition.
A recently reported reader study documents that PI-RADS 2.0 enables prostate cancer detection with average sensitivity of 63%, across all lesions and all readers (including both general radiologists and subspecialists in prostate MRI), with a high index of 74% of specific agreement among readers.16 Another recent study from a data warehouse including four million patients in the Chicago area suggested a local increase of 486% in the use of mpMRI for detection and management of prostate cancer from 2013 to 2015.18
Diffusion-weighted imaging sequences are sensitive to random water motion in tissues. Diffusion is typically restricted in tumors compared to normal tissue. High b-value imaging used to make DWI sequences of prostate cancer show high signal in tumor tissue. Apparent-diffusion coefficient maps are used to quantify the restriction of diffusion (dark signal) by measuring at multiple b-values (i.e. gradient amplitudes). Values of ADC are decreased with restricted diffusion, but this is non-specific. (Figures 3 and 4).19 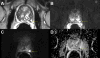 | Figure 3 Multiparametric MRI of a Typical Malignant Prostate Nodule in a 61-Year-Old Male |
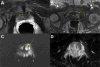 | Figure 4 Multiparametric MRI of the Prostate of a 65-Year-Old Man |
Multiparametric MRI-US Fusion Multiparametric MRI is more sensitive and specific than TRUS, but it is more expensive, more time-consuming, and more uncomfortable than TRUS, requires non-ferromagnetic equipment, and lacks the real-time feedback of TRUS. In order to optimize the advantages of both modalities, mpMRI-US fusion guided biopsies were developed in which previously acquired MRI scans are mapped onto TRUS using co-registration or fusion techniques that employ electromagnetic sensors.
Using technology similar to GPS positioning systems, the latest commercially available devices use three-dimensional (3D) volumetric US acquisitions with either rigid or elastic fusion of the superimposed 3D volumetric MRI. Rigid fusion permits better co-registration between the two modalities, while elastic fusion enables localized corrections for deformations in shape due to positioning, bladder and rectal filling, and different degrees of compression from the endorectal balloon compared with the TRUS probe. Thereafter, significant lesions are mapped and targeted for mpMRI-TRUS fusion guided biopsies (Figure 5).19–26 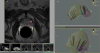 | Figure 5 Multiparametric MRI-TRUS Fusion Guided Biopsy in a 62-Year-Old Male |
Limitations of this technique include imprecise co-registration, inability to compensate for motion or prostate compression causing registration error during biopsy, and requirements for expertise during needle positioning and deployment. The cost of mpMRI-TRUS fusion and its reimbursement, limited availability, variety of fusion equipment and techniques, and lack of uniform guidelines are problematic at present. Harmonizing these two last-mentioned factors will empower a clear assessment of the clinical utility of the technique.27,28
Multiparametric MRI-TRUS fusion directed biopsy increases the detection rate of clinically significant prostate cancer and decreases that of clinically insignificant cancers. The grading and staging are more accurate than using TRUS guidance alone.14,24 A meta-analysis of 15 studies with 2,293 patients showed that the median detection rate for clinically significant disease was about 33%, while that of standard TRUS was 24%.29 Another meta-analysis of 11 studies and 2,626 patients showed a similar higher cancer detection rate with fusion.30 There is still a significant false negative rate with this fusion technique, i.e. as high as 20% in patients when no significant lesions are found on fusion imaging.31–33 However, a recent report found that only 62 of 1,003 cases (6.2%) were upgraded by systematic TRUS biopsy after fusion biopsy, indicating that fusion biopsy infrequently misses important cases.27 |
The PET/CT imaging modality is a well-known hybrid fusion imaging technique that marries together the excellent spatial resolution of computed tomography with functional imaging that highlights metabolic activity in abnormal cells. Whole-body PET/CT has become a mainstay of oncologic imaging because it can localize and quantitate active tumor metabolism at the primary site, in metastases, and in local and distant recurrence, as well as guide tissue sampling and medical decision-making about therapy.
Recently PET/MR hybrid imaging devices have become commercially available, offering the advantages of high specificity of PET with better soft tissue contrast and intrinsic functional MR information compared to PET/CT. However, PET/MR equipment is very expensive, with relatively slow operating speed, and remains to be validated with clinical research.34
There are a number of PET probes that are under investigation for prostate cancer imaging, including 11C-choline, 18F-fluoroethylcholine, 11C-methionine, and 18F-dihydrotestosterone. The specificity needed to differentiate prostate cancer from prostatitis or benign prostatic hypertrophy and the desire for improved sensitivity of PET with its limited resolution are possible barriers to success of these agents.
A very promising imaging biomarker for prostate cancer is PSMA. After 68Ga PSMA is injected into the patient, it binds to cells that express PSMA. The antigen is a transmembrane protein that is overexpressed by prostate cancer cells up to 1000-fold compared to normal prostatic tissue.35 It is noteworthy that increasingly aggressive prostate cancers have increased expression of PSMA.36
However, PSMA is also expressed in other normal tissues such as salivary glands, bladder, pancreas, lung, kidneys, lacrimal glands, liver, spleen, intestines, celiac ganglion, and astrocytes.37–39 It is also seen in duodenal mucosa, some proximal renal tubule cells, some neuroendocrine cells in colon crypts, and some transitional cell, renal cell, colon, and other carcinomas.37 False negative scans have been seen in prostate cancer that has neuroendocrine differentiation, small size lesions, and lesions in close proximity to high physiologic uptake such as the celiac ganglia.40
The 68Ga PSMA PET/CT (or PET/MR) hybrid modality may provide localization staging of primary prostate cancer, guide biopsy, and even offer future opportunities for focal therapy through theranostics. This agent outperforms 18F-choline and 11C-choline in primary staging and restaging of prostate cancer.41,42 Gallium-68 is available using gallium generators which may be located in the imaging department without the need for access to a cyclotron,43 and has a relatively short half-life of 68 minutes. It is quickly cleared and has relatively low background activity.44
There are early reports of accurate primary local staging and improved detection of metastatic lymphadenopathy with high sensitivity and specificity using PSMA PET/CT.41,45–50 [68Ga]Gallium PSMA used with PET/CT outperformed standard CT and MRI in one study of 130 patients with a sensitivity of 75%, specificity of 98.9%, and accuracy of 88.5% compared to 43.9%, 85.4%, and 72.3%, respectively, for CT and MRI.41 Similar findings were noted in a study of 37 patients with intermediate- or high-risk prostate cancer, with sensitivity of 75% and specificity 96% with the same agent.51 Another study of 21 patients showed moderate sensitivity of 67%, specificity of 92%, positive predictive value of 97%, negative predictive value of 42%, and 72% accuracy using a six segment model (Figure 6).52 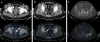 | Figure 6 A 59-Year-Old Male with Newly Diagnosed Prostate Cancer (Gleason Score, 7) |
However, there is a false negative rate of PSMA imaging, reportedly 8% of patients in one study.41 In another study, two of four negative scans were false negatives.51
There is speculation that the negative studies may be due to a saturation effect of uptake from the primary cancer in the prostate gland, or micrometastases below a threshold size for detection, or due to inadequate sample size in one cohort of 30 patients. That same study reported PSMA PET/CT results for detection of nodal metastases with a sensitivity of 33.3%, specificity of 100%, positive predictive value 100%, and negative predictive value 100%.49 The importance of detecting micrometastases cannot be overemphasized because nodal involvement affects staging and therefore treatment selection. Moreover, the majority of metastatic prostate cancer nodes measure less than 8 mm, such that CT and MRI based on size criteria are known to be inaccurate.53
Now in its infancy, PSMA PET/CT fused imaging will require standards for imaging protocols, reviewing, and reporting that are under development.54
Multimodality Fusion Multimodality fusion of TRUS, mpMRI, and PSMA PET/CT can be accomplished with co-registration of these multiple data sets using dedicated DICOM (Digital Imaging and Communications in Medicine) software platforms. The potential value of such combined imaging is much greater than the sum of its parts. Initially there will be much attention paid to discriminatory information such as improved detection of clinically significant cancers without overdiagnosis, and detection of extracapsular extension, because these findings change management and therapy.
Imaging with 68Ga PSMA PET/CT can augment mpMRI by showing increased activity that is very specific and sensitive for prostate cancer, especially because MRI alone suffers from false positive results and may not detect central gland lesions with the same sensitivity as peripheral ones, as described above (Figure 7). 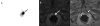 | Figure 7 A 66-Year-Old Male with Newly Diagnosed Prostate Cancer (Gleason Score, 7) |
Multimodality fusion of 68Ga PSMA PET/CT with mpMRI could provide complete local staging of prostate cancer, identification of lymphadenopathy, and evaluation of distant metastases unified into a single imaging display. Add to this the easy access and flexibility of TRUS, also fused with the above, and the advantage of optimized targeted biopsies for primary cancer staging is clear. The opportunity immediately to locate, stage, and treat the tumor is attractive, especially because medical decision-making is streamlined when there is a “one-stop-shop.”
Multiple registration tasks are required for our research: mpMRI with 68Ga PSMA PET/CT, followed by co-registration with real-time TRUS for targeted biopsies. Thereafter, focal therapy guided by the multimodality fusion imaging with HiFUS for focal therapy in organ-confined disease is planned. The ideal reference standard is whole mount prostate pathology sections with histologic mapping onto fusion imaging in patients undergoing radical prostatectomy. We will also track localized needle biopsy results in patients excluded from radical surgery.
Focal Therapy with HiFUS Multimodality imaging has strategic value in planning and guiding focal prostate cancer therapy. Successful focal therapy necessarily involves accurate definition of organ-confined malignancies followed by their complete eradication while sparing surrounding normal tissue. Various methods for focal therapy include high-intensity focused ultrasound (HiFUS), radiofrequency ablation, cryoablation, and electroporation ( Figure 8).
High-intensity focused US uses a sharply focused delivery of high thermal energy to heat and destroy the intended target without ionizing radiation.55,56 Complications have been reported from HiFUS such as bladder outlet obstruction, impotence, urethral stricture, and urinary incontinence.57 However, the potential advantages include reduced morbidity, lower complication rate (impotence and incontinence), and rapid recovery compared to radical surgery. A recent meta-analysis of 13 studies in 346 patients undergoing HiFUS for focal therapy with median follow-up of 12 months showed probability of transition to secondary local treatment was 7.8%, disease-specific survival was 100%, pad-free continence was achieved in 100%, and impotence occurred in 11.4%.58
Several countries have approval for the use of HiFUS devices for human use including the United States, Canada, Australia, and others, with various clinical trials underway. Because there is a need for long-term follow-up and standardization of treatment protocols in using HiFUS for focal therapy,59 this procedure is experimental. It has mostly been used in clinical trials, or in men with comorbidities who have low- to intermediate-risk disease and decline active surveillance or radiation treatment.57,60 |
We hypothesize that multimodality fusion will accurately target clinically significant lesions, improve performance in lesions with Gleason heterogeneity, allow documentation of biopsy locations, and facilitate focal therapy planning and performance. Precision in treating targeted tissues with minimally invasive techniques is the goal. There is a need for speedy fusion with stable registration and accuracy in lesion ablation for successful diagnosis and treatment. Single-center pioneering studies for multimodality prostate cancer imaging and focal therapy, such as the one our institution embarks upon now, may be followed by well-designed prospective multi-center trials.
How can we process the enormous data sets from multimodality imaging? As technical advances continue, we will be pushed to our human limits to process these giant data sets. The answer lies in machine learning using texture analysis with computer-aided detection and diagnosis. Mathematical models and algorithms are a necessity to manage the imaging and clinical data for personalized care. Validation of these models is essential if we are to achieve accurate lesion characterization, risk stratification, staging, treatment selection, surveillance, and evaluation of response to therapy.
This unified approach will grow as we move closer and closer to the holy grail: the ultimate “find it and fix it” using a single theranostic agent that will simultaneously show and kill cancer. Early research with new agents under development shows promise.61,62 |
Magnetic resonance imaging provides the most useful information of the local extent of tumor in prostate cancer, but MRI underestimates lymph node involvement due to the use of short-axis node measurements of 1.0 cm as the criterion for positive nodes. Micrometastases (nodes smaller than 1.0 cm) can be missed.53 A more accurate method of detecting nodal metastases is desirable to achieve accurate preoperative staging.
In patients who do not undergo radical prostatectomy, additional biopsy procedures are essential when extraprostatic tumor may be present to document pathologic stage and triage patients to appropriate therapy. For evaluation of metastases, traditionally bone scan with technetium-99m has been sensitive for detection of bone lesions, while CT or MRI can be accurate for identification of visceral metastases.63
It is noteworthy that PSMA PET/CT also holds great promise as an agent for identification of nodal and bony metastases from prostate cancer. Thus PSMA PET/CT with multimodality fusion imaging may provide not only local staging and targeted prostate biopsies but also may identify extraprostatic sites for selected biopsies to confirm metastases.41 In cases with documented metastases, inappropriate focal therapy can be avoided. |
There are many challenges to overcome. Regulatory requirements, funding for expensive research and development of experimental agents, and undefined reimbursement are but a few. Nevertheless, multimodality imaging and focal therapy for prostate cancer based on future well designed multi-center prospective clinical trials will probably inform clinical decision-making for decades to come. |
3D |
three-dimensional |
ADC |
apparent-diffusion coefficient |
CT |
computed tomography |
DCE |
dynamic contrast-enhanced |
DICOM |
Digital Imaging and Communications in Medicine |
DWI |
diffusion-weighted imaging |
HiFUS |
high-intensity focused ultrasound |
mpMRI |
multiparametric MRI |
MRI |
magnetic resonance imaging |
PET |
positron emission tomography |
PI-RADS 2.0 |
Prostate Imaging Reporting and Data System Version 2 |
PSMA |
prostate-specific membrane antigen |
TRUS |
transrectal ultrasound |
US |
ultrasound. |
|
|
1. American Cancer Society. Cancer Facts & Figures 2017. Atlanta, GA, USA: American Cancer Society; 2017 [accessed April 10, 2017 ]. Available at: http://bit.ly/2shaX7t. 2. American Cancer Society. Global Cancer Facts & Figures. 3rd Edition. Atlanta, GA, USA: American Cancer Society; 2015 [accessed April 10, 2017 ]. Available at: http://bit.ly/2shyPrt. 3. Draisma G, Etzioni R, Tsodikov A, et al. Lead time and overdiagnosis in prostate-specific antigen screening: importance of methods and context. J Natl Cancer Inst. 2009;101:374–83. https://doi.org/10.1093/jnci/djp001. 4. Moyer VA. US Preventive Services Task Force. Screening for prostate cancer: U.S. Preventive Services Task Force recommendation statement. Ann Intern Med. 2012;157:120–34. 5. Fisher B, Redmond C, Poisson R, et al. Eight-year results of a randomized clinical trial comparing total mastectomy and lumpectomy with or without irradiation in the treatment of breast cancer. N Engl J Med. 1989;320:822–8. https://doi.org/10.1056/NEJM198903303201302. 6. Salami S, Ben-Levi E, Yaskiv O. In patients with a previous negative prostate biopsy and a suspicious lesion on magnetic resonance imaging, is a 12-core biopsy still necessary in addition to a targeted biopsy? BJU Int. 2015;115:562–70. https://doi.org/10.1111/bju.12938. 7. Zhang J, Xiu J, Dong Y, et al. Magnetic resonance imaging-directed biopsy improves the prediction of prostate cancer aggressiveness compared with a 12-core transrectal ultrasound-guided prostate biopsy. Mol Med Rep. 2014;9:1989–97. https://doi.org/10.3892/mmr.2014.1994. 9. Pepe P, Dibenedetto G, Pennisi M, Fraggetta F, Colecchia M, Aragona F. Detection rate of anterior prostate cancer in 226 patients submitted to initial and repeat transperineal biopsy. Urol Int. 2014;93:189–92. https://doi.org/10.1159/000358494. 10. Loch T, Eppelman U, Lehmann J, Wullich B, Loch A, Stöckle M. Transrectal ultrasound guided biopsy of the prostate: random sextant versus biopsies of sono-morphologically suspicious lesions. World J Urol. 2004;22:357–60. https://doi.org/10.1007/s00345-004-0462-4. 11. Taira AV, Merrick GS, Galbreath RW, et al. Performance of transperineal template-guided mapping biopsy in detecting prostate cancer in the initial and repeat biopsy setting. Prostate Cancer Prostatic Dis. 2010;13:71–7. https://doi.org/10.1038/pcan.2009.42. 14. Siddiqui MM, Rais-Bahrami S, Truong H, et al. Magnetic resonance imaging/ultrasound-fusion biopsy significantly upgrades prostate cancer versus systematic 12-core transrectal ultrasound biopsy. Eur Urol. 2013;64:713–19. https://doi.org/10.1016/j.eururo.2013.05.059. 15. Muller BG, Shih JH, Sankineni S, et al. Prostate cancer: interobserver agreement and accuracy with the revised prostate imaging reporting and data system at multiparametric MR imaging. Radiology. 2015;277:741–50. https://doi.org/10.1148/radiol.2015142818. 16. Greer MD, Brown AM, Shih JH, et al. Accuracy and agreement of PIRADSv2 for prostate cancer mpMRI: a multireader study. J Magn Reson Imaging. 2017;45:579–85. https://doi.org/10.1002/jmri.25372. 17. American College of Radiology. PI-RADS™. Prostate Imaging – Reporting and Data System, 2015 version 2. [accessed April 12, 2017 ]. Available at: http://bit.ly/2tow9bB. 18. Oberlin DT, Casalino DD, Miller FH, Meeks JJ. Dramatic increase in the utilization of multiparametric magnetic resonance imaging for detection and management of prostate cancer. Abdom Radiol. 2017;42:1255–8. https://doi.org/10.1007/s00261-016-0975-5. 19. Turkbey B, Brown AM, Sankineni S, Wood BJ, Pinto PA, Choyke PL. Multiparametric prostate magnetic resonance imaging in the evaluation of prostate cancer. CA Cancer J Clin. 66:326–36. 216. https://doi.org/10.3322/caac.21333. 20. Wysock JS, Rosenkrantz AB, Huang WC, et al. A prospective, blinded comparison of magnetic resonance (MR) imaging-ultrasound fusion and visual estimation in the performance of MR-targeted prostate biopsy: the PROFUS trial. Eur Urol. 2014;66:343–51. https://doi.org/10.1016/j.eururo.2013.10.048. 21. Kasivisvanathan V, Dufour R, Moore CM, et al. Transperineal magnetic resonance image targeted prostate biopsy versus transperineal template prostate biopsy in the detection of clinically significant prostate cancer. J Urol. 2013;189:860–6. https://doi.org/10.1016/j.juro.2012.10.009. 22. Yerram NK, Volkin D, Turkbey B, et al. Low suspicion lesions on multiparametric magnetic resonance imaging predict for the absence of high-risk prostate cancer. BJU Int. 2012;110(11 pt B):E783–8. https://doi.org/10.1111/j.1464-410X.2012.11646.x. 23. Sonn GA, Chang E, Natarajan S, et al. Value of targeted prostate biopsy using magnetic resonance-ultrasound fusion in men with prior negative biopsy and elevated prostate-specific antigen. Eur Urol. 2014;65:809–15. https://doi.org/10.1016/j.eururo.2013.03.025. 24. Siddiqui MM, Rais-Bahrami S, Turkbey B, et al. Comparison of MR/ultrasound fusion-guided biopsy with ultrasound-guided biopsy for the diagnosis of prostate cancer. JAMA. 2015;313:390–7. https://doi.org/10.1001/jama.2014.17942. 25. Watson MJ, George AK, Maruf M, et al. Risk stratification of prostate cancer: integrating multiparametric MRI, nomograms and biomarkers. Future Oncol. 2016;12:2417–30. https://doi.org/10.2217/fon-2016-0178. 26. Sankineni S, George AK, Brown AM, et al. Posterior subcapsular prostate cancer: identification with mpMRI and MRI/TRUS fusion-guided biopsy. Abdom Imaging. 2015;40:2557–65. https://doi.org/10.1007/s00261-015-0426-8. 27. Muthigi A, George AK, Sidana A, et al. Missing the mark: prostate cancer upgrading by systematic biopsy over magnetic resonance imaging/transrectal ultrasound fusion biopsy. J Urol. 2017;197:327–34. https://doi.org/10.1016/j.juro.2016.08.097. 29. Valerio M, Donaldson I, Emberton M, et al. Detection of clinically significant prostate cancer using magnetic resonance imaging-ultrasound fusion targeted biopsy: a systematic review. Eur Urol. 2015;68:8–19. https://doi.org/10.1016/j.eururo.2014.10.026. 30. Gayet M, van der Aa A, Beerlage HP, Schrier BP, Mulders PF, Wijkstra H. The value of magnetic resonance imaging and ultrasonography (MRI/US)-fusion biopsy platforms in prostate cancer detection: a systematic review. BJU Int. 2016;117:392–400. https://doi.org/10.1111/bju.13247. 31. Wysock JS, Mendhiratta N, Zattoni F, et al. Predictive value of negative 3T multiparametric magnetic resonance imaging of the prostate on 12 core biopsy results. BJU Int. 2016;118:515–20. https://doi.org/10.1111/bju.13427. 32. Filson CP, Natarajan S, Margolis DJ, et al. Prostate cancer detection with magnetic resonance-ultrasound fusion biopsy: the role of systematic and targeted biopsies. Cancer. 2016;122:884–92. https://doi.org/10.1002/cncr.29874. 34. Wang SY, Chen XX, Li Y, Zhang YY. Application of multimodality imaging fusion technology in diagnosis and treatment of malignant tumors under the precision medicine plan. Chin Med J (Engl). 2016;129:2991–7. https://doi.org/10.4103/0366-6999.195467. 37. Silver DA, Pellicer I, Fair WR, Heston WD, Cordon-Cardo C. Prostate-specific membrane antigen expression in normal and malignant human tissues. Clin Cancer Res. 1997;3:81–5. 38. Afshar-Oromieh A, Malcher A, Eder M, et al. PET imaging with a [ 68Ga]gallium-labelled PSMA ligand for the diagnosis of prostate cancer: biodistribution in humans and first evaluation of tumor lesions. Eur J Nucl Med Mol Imaging. 2013;40:486–95. https://doi.org/10.1007/s00259-012-2298-2. 40. Chakraborty PS, Tripathi M, Agarwal KK, Kumar R, Vijary MK, Bal C. Metastatic poorly differentiated prostate carcinoma with neuroendocrine differentiation: negative on 68Ga-PSMA PET/CT. Clin Nucl Med. 2015;40:e163–6. https://doi.org/10.1097/RLU.0000000000000594. 41. Maurer T, Gschwend JE, Rauscher I, et al. Diagnostic efficacy of 68Gallium-PSMA positron emission tomography compared to conventional imaging in lymph node staging of 130 consecutive patients with intermediate to high risk prostate cancer. J Urol. 2016;195:1436–43. https://doi.org/10.1016/j.juro.2015.12.025. 43. Rowe SP, Drzezga A, Neumaier B, et al. Prostate-specific membrane antigen-targeted radiohalogenated PET and therapeutic agents for prostate cancer. J Nuc Med. 2016;57:90S–6S. https://doi.org/10.2967/jnumed.115.170175. 44. Eder M, Schäfer M, Bauder-Wüst U, et al. 68Ga-Complex lipophilicity and the targeting property of a urea-based PSMA inhibitor for PET imaging. Bioconjugate Chem. 2012;23:688–97. https://doi.org/10.1021/bc200279b. 45. Zamboglou C, Schiller F, Fechter T, et al. 68Ga-HBED-CC-PSMA PET/CT versus histopathology in primary localized prostate cancer: a voxel-wise comparison. Theranostics. 2016;6:1619–28. https://doi.org/10.7150/thno.15344. 46. Zamboglou C, Drendel V, Jilg CA, et al. Comparison of 68Ga-HBED-CC PSMA-PET/CT and multiparametric MRI for gross tumour volume detection in patients with primary prostate cancer based on slice by slice comparison with histopathology. Theranostics. 2017;7:228–37. https://doi.org/10.7150/thno.16638. 47. Kabasakal L, Demirci E, Ocak M, et al. Evaluation of PSMA PET/CT imaging using a 68Ga-HBED-CC ligand in patients with prostate cancer and the value of early pelvic imaging. Nucl Med Commun. 2015;36:582–7. https://doi.org/10.1097/MNM.0000000000000290. 49. Budäus L, Leyh-Bannurah SR, Salomon G, et al. Initial experience of 68Ga-PSMA PET/CT imaging in high-risk prostate cancer patients prior to radical prostatectomy. Eur Urol. 2016;69:393–6. https://doi.org/10.1016/j.eururo.2015.06.010. 50. Rahbar K, Weckesser M, Huss S, et al. Correlation of intraprostatic tumor extent with 68Ga-PSMA distribution in patients with prostate cancer. J Nucl Med. 2016;57:563–7. https://doi.org/10.2967/jnumed.115.169243. 51. Eiber M, Maurer T, Beer AJ, et al. Prospective evaluation of PSMA-PET imaging for preoperative lymph node staging in prostate cancer [Abstract]. J Nuc Med. 2014;55(Suppl 1):20. 53. Hövels AM, Heesakkers RA, Adang EM, et al. The diagnostic accuracy of CT and MRI in the staging of pelvic lymph nodes in patients with prostate cancer: a meta-analysis. Clin Radiol. 2008;63:387–95. https://doi.org/10.1016/j.crad.2007.05.022. 54. Rauscher I, Maurer T, Fendler WP, Sommer WH, Schwaiger M, Eiber M. 68GA-PSMA ligand PET/CT in patients with prostate cancer: how we review and report. Cancer Imaging. 2016;16:14. https://doi.org/10.1186/s40644-016-0072-6. 56. Warmuth M, Johansson T, Mad P. Systematic review of the efficacy and safety of high intensity focused ultrasound for the primary and salvage treatment of prostate cancer. Eur Urol. 2010;58:803–15. https://doi.org/10.1016/j.eururo.2010.09.009. 57. Mottet N, Bellmunt J, Bolla M, et al. EAU-ESTRO-SIOG guidelines on prostate cancer. Part 1: Screening, diagnosis, and local treatment with curative intent. Eur Urol. 2017;71:618–29. https://doi.org/10.1016/j.eururo.2016.08.003. 61. Sterzing F, Kratochwil C, Fiedler H, et al. 68Ga-PSMA-11 PET/CT: a new technique with high potential for the radiotherapeutic management of prostate cancer patients. Eur J Nucl Med Mol Imaging. 2016;43:34–41. https://doi.org/10.1007/s00259-015-3188-1. 63. Buyyounouski MK, Choyke PL, McKenney JK, et al. Prostate cancer – major changes in the American Joint Committee on Cancer eighth edition cancer staging manual. CA Cancer J Clin. 2017;67:245–53. https://doi.org/10.3322/caac.21391. |