| |
|
RMMJ
Rambam Maimonides Medical Journal
Rambam Health Care Campus 2024; 15(3): e0015.
ISSN: 2076-9172 Published online 2024 July 30. doi: 10.5041/RMMJ.10529
Navigating the Complexity of Alternating Hemiplegia in Childhood: A Comprehensive Review Jamir Pitton Rissardo, M.D,1* Nilofar Murtaza Vora, M.D.,2 Yogendra Singh, M.D.,2 Sweta Kishore, M.D.,2 and Ana Letícia Fornari Caprara, M.D.3 1Neurology Department, Cooper University Hospital, Camden, New Jersey, USA 2Medicine Department, Terna Speciality Hospital and Research Centre, Navi Mumbai, India 3Medicine Department, Federal University of Santa Maria, Santa Maria, Brazil
|
Alternating hemiplegia of childhood (AHC) is a complex neurodevelopmental disorder characterized by paroxysmal and transient events of unilateral or bilateral paresis, usually occurring before 18 months of age. Mutations in the ATP1A3 gene, mainly p.Asp801Asn, p.Glu815Lys, and p.Gly947Arg at the protein level, are found in around 80% of the individuals with AHC. Interestingly, these mutations reflect the degree of severity of the neurological symptoms (p.Glu815Lys > p.Asp801Asn > p.Gly947Arg). Some channels involved in this disorder are N-type voltage-gated calcium channels, ATP-sensitive potassium channels, and the sodium/calcium exchanger. In this context, the management of AHC should be divided into the treatment of attacks, prophylactic treatment, and management of comorbidities commonly found in this group of individuals, including epilepsy, attention-deficit/hyperactivity disorder, aggressive behavior, cognitive impairment, movement disorders, and migraine. The importance of an integrated approach with a multidisciplinary team, such as neuropsychologists and dietitians, is worth mentioning, as well as the follow-up with a neurologist. In the present study, we propose new diagnostic criteria for AHC, dividing it into clinical, laboratory, supporting, and atypical features. Also, we review the location of the mutations in the ATP1A3 protein of individuals with AHC, rapid-onset dystonia-parkinsonism (RDP) variants, and early infantile epileptic encephalopathy (variants with hemiplegic attack). We also include a section about the animal models for ATP1A3 disorders.
Keywords: Aicardi, alternating hemiplegia of childhood, ATP1A2, ATP1A3, flunarizine, rare disease |
Alternating hemiplegia of childhood (AHC) is a complex neurodevelopmental disorder mainly characterized by paroxysmal transient events of unilateral or bilateral paresis, usually before 18 months.1 It is a rare and severe neurological disorder with a prevalence of 1:100,000 to 1:1,000,000.2 Little is known about the several genetic, environmental, and biological factors that could potentially contribute to the pathophysiology of AHC. In this way, diagnosis and management are empirical in many cases, with few evidence-based studies to support specific tests or therapeutic strategies.
The AHC disorder has been associated with mutations in the ATP1A3 gene, which encodes the alpha-3 subunit of the neuronal Na+/K+-ATPase transmembrane ion pump in approximately 75% of patients.3 The ATP1A3 gene is highly expressed in brain regions that influence the autonomic nervous system.4 In approximately a quarter of the patients, ATP1A2 mutations were encountered, while in a minority of the subjects, the etiology is still unknown.
The presentation of AHC is often associated with precipitating factors such as environmental and psychological stressors. Paroxysmal episodes may occur independently or in association with other clinical manifestations, such as autonomic dysfunction, altered mental status, and abnormal movements, such as dystonia, ataxia, and choreoathetosis.5
A typical clinical pattern can be observed in most individuals with AHC. In this way, it is possible to divide the clinical progression of the disease into three phases. The first phase occurs during the first 3 months of age, and the main clinical feature is abnormal ocular movements, which can be associated with dystonia.1 The second phase generally starts at 4 months and usually extends until 6 years; it is characterized by hemiplegic spells. In this phase, the patient can also present delayed developmental milestones and seizures.5 In the last phase, unremitting neurological deficits could be observed. Other significant findings in the last phase include persistent developmental delays and, less frequently, hemiplegic and dystonic spells.6
Patients with AHC have a significant burden of neurological comorbidities and frequently also have deficits in sustained attention, reduced speed of information processing, and difficulties in understanding, speaking, and with working memory.7 Moreover, patients often have gross motor function impairments, which can be complicated by attention-deficit/hyperactivity disorder, disruptive behavior, and anxiety disorders.8 Interestingly, some clinical manifestations occur in distinctive and sequential phases, disappearing during the sleep cycle.9
Advanced molecular research has allowed a better understanding of the main genes involved in this condition and contributes to early confirmation of the diagnosis. In this way, the present study aims to review the recent advancements in understanding the clinical features and pathophysiology of AHC, as well as current recommendations for genetic testing and treatment strategies. |
Onset and Frequency Usually, AHC episodes start before 18 months of age and are generally related to specific triggers. The most common early clinical signs include autonomic dysfunctions associated with precipitating factors, ocular abnormalities, and tonic or dystonic episodes. Paroxysmal tonic or dystonic episodes frequently occur in one limb or, less frequently, a hemibody. The most common type of ocular abnormality reported is nystagmus, which usually affects only one eye and can manifest alone or in conjunction with dystonic or tonic symptoms. 10
Hemiplegia Hemiplegia is the central and most prominent symptom of AHC. It involves a temporary loss of motor function on one side of the body during an episode. The paralysis can be partial or complete and is reversible, typically resolving once the episode subsides. 1 The alternating pattern of hemiplegia is a distinguishing characteristic of AHC. This alternating paralysis can affect various body parts, including the face, arms, and legs. The hemiplegic episodes in their classical presentations are mild and irregular when they first start, but they eventually evolve into a characteristic clinical appearance. 8 Plegic attacks can affect one side or, less often, both sides and can begin suddenly or develop gradually over several minutes. Episodes can last from a few minutes to several days and occur at varying frequencies. 9 During an attack, the patient often maintains consciousness but is restless and anxious. Usually, the lower limbs are not as severely affected as the arms. Hemiplegia can alternate from side to side in the same episode. 5
Motor Symptoms During hemiplegic episodes, there is a significant impairment of motor function on the affected side. This can manifest as weakness, stiffness, and difficulty in voluntary movements. Motor milestone delays are commonly reported. Bourgeois noted that 24 participants had poor fine-motor control and had only learned to walk independently at the average age of 44 months. Often, the first gross motor abnormality to be reported is hypotonia. 11 Another study by Masoud et al. examined the functions of gross motor, upper extremity motor control, motor speech, and dysphagia in 23 AHC patients; 9 were males, and 14 were females, with a mean age of 9.33 years. 12 Their findings indicated that speech impairments related to motor function were markedly more severe than gross motor abnormalities as determined by the Gross Motor Function Classification System (GMFCS). Only three patients were defined as moderate to severe according to the GMFCS criteria. Also, Masoud et al. recognized that the oropharyngeal function is one of the most severely impaired functional domains in patients with AHC. 12
Oculomotor Disturbances The AHC disorder is associated with distinct oculomotor disturbances, encompassing several eye movement abnormalities. Nystagmus is frequently observed, but strabismus may also be present. These eye abnormalities can be exacerbated during episodes of hemiplegia, contributing to the complexity of the clinical presentation. Aicardi et al. reported a cohort of 20 patients, in which nystagmus was observed in 90%. The nystagmus was characterized as monocular in 14, horizontal in 13, and vertical in 3 patients. 10 The nystagmus and hemiplegia typically alternate from eye to eye with each subsequent episode. 10, 13 Anisocoria, or a larger pupil contralateral to the abducting eye, was rarely described. 14 During the spells, the non-abducting eye has been reported to have reduced horizontal but intact vertical movements. 15 Interestingly, some patients can present horizontal abduction jerks of one eye. Still, the abnormal movements of the limbs can be accompanied by a tonic abducting deviation of the eye located on the contralateral side. When vertical nystagmus was observed, it affected both eyes with similar amplitudes. 16
Seizures Seizures occur in a variety of forms, affecting a significant percentage of people with AHC. The most common seizure types in this population are atonic, focal, or generalized tonic–clonic seizures. In AHC, seizures can occur separately or with hemiplegic episodes. For these patients, seizures can be a major source of distress and stigmatization, contributing to social isolation and decreased quality of life, and also leading to an increased number of plegic attacks. Sweney et al. reported generalized, tonic, or tonic–clonic seizures in 44 out of 103 (43%) AHC patients, with the mean age of epileptic onset attacks being around the age of 6 years. 5 Ten children (23%) did not have another seizure until they were 10 years old or older. 5
Cognitive and Developmental Challenges Cognitive impairments are often seen in AHC, including learning difficulties, intellectual disability, and developmental delays. Additionally, Mikaki et al. observed delayed developmental milestones in 40 out of 44 (90%) of the patients with AHC. Also, they noted a correlation between cognitive impairment and the age of hemiplegic attack onset in these individuals. 1 Therefore, the findings reported by Mikaki et al. raised questions about whether the cognitive impairment seen in people with AHC is a consequence of the neurological condition or a symptomatic manifestation of the paroxysmal episodes. 1
Autonomic Dysfunction Some individuals with AHC experience autonomic dysfunction, which can manifest as problems in regulating heart rate, gastric emptying, and body temperature. The hypothalamus and vagus nerve nuclei, which regulate the autonomic nervous system, have high expression levels of ATP1A3. 4, 17 Additionally, this gene is expressed in gamma-aminobutyric acid interneurons that can regulate motility and in the motor brain stem nuclei that regulate swallowing. 18
Sleep Disturbances An adequate sleep cycle has been shown to have a significant clinical benefit in managing plegic attacks, and sleep dysfunction has been observed in patients with AHC. 10, 11 Individuals with AHC often exhibit irregular sleep patterns. The alternating hemiplegic episodes, which characterize the condition, can disrupt the normal sleep–wake cycle, leading to inconsistencies in sleep duration and quality noticed in polysomnography studies. Dysautonomia, a common feature of AHC, can affect the autonomic control of respiration. Irregular respiratory patterns during sleep may impact sleep quality and potentially lead to sleep-related breathing disorders. A study by Kansagra et al. found that 20 of 22 patients with AHC had at least one type of sleep problem, of which 6 had obstructive sleep apnea syndrome, a high mean overall apnea-hypopnea score, and a high mean arousal index. 6
Neuropsychological Disturbances Jasien et al. evaluated neuropsychological abnormalities in 25 individuals with AHC and found significant impairments in cognition, expressive and receptive language, executive function, attention, and behavior. 19 In this study, 10 patients with AHC showed attention-deficit/hyperactivity disorder, 7 had disruptive behavior, and 3 had anxiety disorder. 19 Eight out of 25 subjects exhibited impairments in comprehension and long-term memory, inadequate academic performance, and dyscalculia. 19 In the same study, cognitive function was normal in 4 (16%), borderline in 3 (12%), and impaired in 18 (72%), of which 6 (24%) were mild, 10 (40%) moderate, and 2 (8%) severe. 19
Migraine Migraine is not commonly reported in patients with AHC, and the prevalence varies widely in the literature. Sakuragawa reported that only 9.1% of the individuals had a family history of migraine, and 13% of the subjects reported headaches as a prodrome of the plegic attacks. 9 Mikati et al. revealed a prevalence of 25% of migraine in immediate family members of individuals with AHC. 1 Saito et al. reported a single case of migraine out of 10 in patients with AHC. 20 |
ETIOLOGY AND PATHOPHYSIOLOGY
Genetic Basis Alternating hemiplegia of childhood is primarily associated with mutations in the ATP1A3 gene on chromosome 19q13. 21 This gene encodes the alpha-3 subunit of the Na +/K +-ATPase pump. 22 Although AHC is a sporadic disorder caused by de novo variants, a few autosomal dominant inherited cases have been reported ( Figure 1). 23– 26 Germline mosaicism has been described in familial cases of other ATP1A3-related disorders but has still not been reported in AHC. 27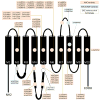 | Figure 1 Location of Alternating Hemiplegia of Childhood (AHC) Variants in the ATP1A3 Protein, Including Rapid-onset Dystonia-parkinsonism (RDP) Variants and Early Infantile Epileptic Encephalopathy (EIEE) Variants with Hemiplegic Attack. |
Some of the ATP1A3-related disorders are AHC, rapid-onset dystonia-parkinsonism (RDP), and cerebellar ataxia, areflexia, pes cavus, optic atrophy, and sensorineural hearing loss (CAPOS) syndrome.
Clinical data from a cohort of 155 patients from the International Consortium of AHC were carefully examined, and a distinct genotype-phenotype association study was performed.28 Interestingly, 85% of patients had ATP1A3 mutations, indicating that a small subset fulfilled AHC criteria without any molecular genetic diagnosis. The study also confirmed the incidence of the three most common mutations: p.Asp801Asn, p.Glu815Lys, and p.Gly947Arg. Of these, p.Glu815Lys was associated with a more severe phenotype, with drug-resistant epilepsy, profound intellectual disability, and severe dystonia. The p.Asp801Asn mutation appears to be associated with a milder phenotype with later onset of the paroxysmal events and less frequent plegic attacks; the majority of these patients present moderate intellectual disability with a higher rate of behavioral problems. The presence of p.Gly947Arg correlates with a favorable prognosis. Also, paroxysmal events have a later onset with p.Gly947Arg compared to the other two mutations. Moreover, no severe intellectual disability has been reported with p.Gly947Arg. In conclusion, the three mutations show a gradient of the severity of the symptoms: p.Glu815Lys > p.Asp801Asn > p.Gly947Arg.28
Table 1 lists the percentages of the three most frequent mutations encountered in individuals with AHC in different cohorts (Table 1).3,23,28–36 Prognosis can be reasonably predicted for patients with these recurrent ATP1A3 mutations. However, more cohort studies are needed in the literature for a complete understanding of the best therapeutic choices and clinical course of this rare syndrome. Also, the present cohorts in the literature provide symptoms not commonly seen in individuals with AHC but usually seen in other ATP1A3 disorders. This overlap of clinical manifestations may suggest a continuum spectrum of the disorders related to this genetic mutation. 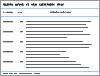 | Table 1 Frequency of Alternating Hemiplegia of Childhood Variants in Different Cohorts. |
Ion Transport Dysfunction The Na +/K +-ATPase pump is fundamental for maintaining the resting membrane potential of neurons ( Figure 2). Disruption of this pump contributes to the hyperexcitability of neurons, abnormal firing patterns, and impaired regulation of neurotransmitter release. The mutations in ATP1A3 result in dysfunctional sodium-potassium pump activity, leading to impaired ion transport and disrupted neuronal excitability. The alpha-3 subunit is present on the cytosolic side of the cell membrane of neurons and glial cells, which explains the neurological findings seen in AHC. 18, 37 In the neuron with the alpha-3 allele mutation, Na +/K +-ATPase has a reduced sodium affinity, resulting in an increased intracellular Na + concentration and a variety of dramatic events such as increased calcium influx into the cell with lethal effects and the liberation of excitatory amino acids. 38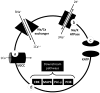 | Figure 2 Schematic Representation of the Downstream Pathways and the Na+-K+-ATPase at the Cell Membrane. |
Different pathogenic causes could explain heterogeneity in the phenotypic range of patients with AHC and RDP. Therefore, based on the description and characterization of ATP1A3 mutations related to AHC, the activity of the Na+/K+-ATPase pump appears to be essential for pathogenicity in AHC.36 As a result, most RDP mutations likely influence protein expression and cell surface expression, but in AHC a change in the pump activity could explain the phenotype.
Environmental and Epigenetic Modifiers The most significant players in the pathophysiology of AHC are probably mutations related to ATP1A3. Still, environmental factors are believed to trigger or exacerbate episodes in genetically susceptible individuals. Physiological or psychological stressors usually trigger the onset of AHC symptoms.
Some triggers already reported in the literature are strenuous exercise, illness, prolonged fasting, and psychological stress. Also, environmental stressors (bright light, excessive heat or cold, noise, crowds), water exposure (bathing and swimming), and certain foods or odors (chocolate and food dyes) are associated with the occurrence of AHC symptoms.39 In this context, the interaction between genes and environmental factors may be responsible for the diverse phenotypes and disease spectrum. |
DIAGNOSIS AND DIFFERENTIAL DIAGNOSIS
Alternating hemiplegia of childhood is a rare disorder first reported in the medical literature in 1971 by Simon Verret and John C. Steele.40 Nevertheless, only two decades later, diagnostic criteria were proposed by Ingeborg Krägeloh-Mann and Jean Aicardi, who also emphasized that AHC was a nosologic entity distinct from hemiplegic migraine.10 There is no diagnostic test for AHC, and it remains a clinical diagnosis. The diagnosis can be obtained according to six clinical criteria, known as the Aicardi criteria.10 One of the cardinal diagnostic criteria of AHC is the remission of hemiplegia and other paroxysmal events, but not seizures, with sleep and their potential recurrence shortly after waking.41 However, in 2012, the molecular abnormality of ATP1A3 was identified as the primary etiology of AHC (Table 2).5,7,23,42,43 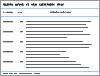 | Table 2 Diagnostic Criteria for Alternating Hemiplegia of Childhood Proposed by Rissardo et al. |
Moyamoya angiopathy and mitochondrial diseases, such as Kearns–Sayre syndrome, mitochondrial encephalomyopathy, lactic acidosis, and stroke-like episodes, are a few disorders with clinical manifestations that may overlap with AHC. Moyamoya angiopathy presents with transient ischemic attack and visual disturbances, aphasia, weakness, numbness, or paralysis involving the face, arm, or leg, typically in one side of the body, mimicking the AHC episodes.44 It is a vascular disorder characterized by progressive stenosis of the terminal portion of the internal carotid arteries and the development of a network of abnormal collateral vessels in which the carotid artery becomes partially or completely occluded, compromising cerebral blood flow. Neuroimaging can help differentiate Moyamoya syndrome from AHC since Moyamoya syndrome could lead to strokes.
The AHC disorder may mimic Kearns–Sayre syndrome, a subtype of chronic progressive external ophthalmoplegia (CPEO). In addition to CPEO symptoms, Kearns–Sayre syndrome usually presents before the age of 20 years with pigmentary retinopathy. Individuals affected may also show other clinical features such as complete heart block, high protein levels in cerebrospinal fluid, cerebellar ataxia, deafness, intellectual delay, and endocrine abnormalities.45
On the other hand, mitochondrial encephalomyopathy, lactic acidosis, and stroke-like episodes present in children or young adults as recurrent episodes of encephalopathy, myopathy, headache, and focal neurologic deficits that slowly progress.41
Clinical differentiation of AHC should include other disorders of the group of ATP1A3-related disorders.46 The disorders that most commonly overlap in clinical manifestations with AHC are RPD and CAPOS syndrome (Figure 3) (Table 3).47 But other conditions should also be considered due to similarities in clinical presentation, such as early infantile epileptic encephalopathy (EIEE), relapsing encephalopathy with cerebellar ataxia, and childhood rapid-onset ataxia.48,49 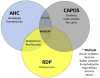 | Figure 3 Overlapping of AHC, CAPOS, and RDP. |
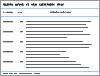 | Table 3 Clinical Characteristics of Alternating Hemiplegia of Childhood (AHC), Cerebellar Ataxia, Areflexia, Pes Cavus, Optic Atrophy, and Sensorineural Hearing Loss (CAPOS) Syndrome, and Rapid-onset Dystonia-Parkinsonism (RDP). |
Benign familial nocturnal alternating hemiplegia of childhood (BNAHC) is considered a probably migraine-related disorder presenting with recurrent attacks of hemiplegia arising from sleep and observed in children in the absence of neurological or cognitive impairment (Table 4).1,6,7,50–53 Maas et al. reported on two cases, and a further 12 cases from the literature, stating that the age of onset of BNAHC ranged from 4 months to 3 years.54 Episodes of hemiplegia occurred during nocturnal or daytime sleep and were associated with inconsolable crying.42 Familial or sporadic hemiplegic headache is a subtype of migraine with aura. Individuals with this disorder experience weakness on one side of the body just before or during migraine headaches, and the degree of weakness can vary from mild to severe. Reports of weakness are also associated with other types of aura and visual symptoms, which may affect only a part of the body and, more rarely, the entire body.55 The broader use of genetic technology has enabled the differentiation of the AHC diagnosis from similar disorders and its clinical spectrum to be extended. 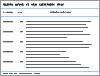 | Table 4 Clinical Features of Atypical Cases of Alternating Hemiplegia of Childhood (AHC). |
|
The prevalence of AHC is 1:1,000,000 in children under the age of 16 years. Still, this number could be underestimated due to the variability in clinical presentation and the lack of genetic analysis in the preceding epidemiologic data.56
One of the largest AHC studies was conducted by Sweney et al., who reported on 103 individuals (56 females and 47 males).5 According to these authors, the most frequent and early symptoms observed in the first 3 months of life were paroxysmal eye movements in 83% and hemiplegic episodes by 6 months in 56% of patients.5 Dystonic symptoms preceded hemiplegic episodes in 35/86 (41%) cases, and both dystonic and plegic episodes had a co-occurrence onset in 30/86 (35%).5
Aicardi et al. summarized the results of 75 AHC subjects previously described in the literature, indicating clinical features and frequency: hemiplegia involving either side (100%); episodes of double hemiplegia/quadriplegia (93%); tonic/dystonic attacks (96%); oculomotor abnormalities (nystagmus/gaze deviation/strabismus) (97%); the remission of symptoms with sleep (94%); developmental delay or intellectual disability (98%); and neurological deficits/choreoathetosis/dystonia (90%).10
Pavone et al. revealed intrafamilial clinical variability in the clinical course and long-term outcomes of AHC in twin sisters. In these twins, clinical manifestations of AHC started in the early days of life with episodes of bath-induced abnormal ocular movements that persisted from the first months to 2 years of age.57 |
Managing Acute Attacks Therapeutic management is complex, and every aspect of AHC should be considered. Although no specific disease-modifying agents have been approved for this condition, flunarizine may help in symptomatic management. Prophylactic treatment and acute management of attacks may be addressed in AHC therapy. 46 While several drugs have been suggested, it has been shown that calcium channel blockers have the highest efficacy. Flunarizine is the most often used medication, with a dosage range of 5–20 mg/day, and 10 mg being the most commonly used. Acute therapy should aim to eliminate or reduce the recognized triggers and encourage sleep hygiene measures. Flunarizine was shown to lessen the duration and intensity of symptoms in some cases; most commonly, it was shown to reduce the duration and severity of hemiplegic bouts. 14, 58
Benzodiazepines and other sleep inductors have already been reported to be effective in managing acute attacks of AHC. One of the hypotheses is that the mechanism of facilitating sleep induction helps decrease the number of attacks since paroxysmal attacks frequently disappear during sleep, although attacks may resume soon after awakening.59
Preventing Episodes by Long-term Medication and Avoiding Triggers Bourgeois et al. treated 17 children with AHC with flunarizine. 60 They found that only one child experienced a significant decrease in the frequency of hemiplegic attacks (by more than 50%) and that nine of the children experienced a significant reduction in the severity and duration of the hemiplegic attacks (from several days to a few hours on average). 60 In a large Japanese cohort with 23 AHC cases, more than 75% of the individuals showed a reduction in the frequency and severity of the attacks after flunarizine therapy. 61 Mikaki et al. showed similar benefits with flunarizine, with which a clinically significant decrease in the frequency and/or severity of AHC attacks was achieved in 21 out of 44 patients. Flunarizine completely prevented hemiplegic attacks in 1 patient (4%), only slightly improved attacks in 2 patients (7%), and had no effect in 4 patients. 1 Sweney et al. observed a reduction in the frequency of dystonic and hemiplegic episodes in 60% of the patients using flunarizine and in 38% with long-term benzodiazepine therapy. 5 Notably, several other therapies have already been reported in the literature for managing AHC, but their effectiveness has not been fully established ( Figure 4). These include topiramate, ketogenic diet, triheptanoin steroid, oral adenosine-5′-triphosphate supplementation, amantadine, memantine, aripiprazole, coenzyme Q, acetazolamide, dextromethorphan, and vagus nerve stimulation. 59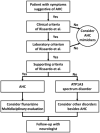 | Figure 4 Management According to the Diagnosis Criteria Proposed by Rissardo et al. |
Neville and Ninan recommend using flunarizine at the beginning of each episode, treating the epileptic seizure, trying to avoid trigger events, and advising adequate sleep.56 Flunarizine is the most frequently prescribed medication for the management of paroxysmal plegic attacks in AHC. Pisciotta et al. observed that flunarizine showed a statistically significant effect in lowering the frequency and duration of paroxysmal attacks in 50% of patients and their intensity in 32%, with considerably increased efficacy related to younger individuals.62
Jiang et al. documented the effectiveness of topiramate in four patients.63 The medication markedly reduced the frequency and duration of hemiplegic episodes in every patient. They also noticed an increased effect in individuals with seizures, migraines, involuntary movements, and autonomic symptoms.63 Therefore, topiramate as a long-term therapeutical choice should be considered for these disorders, when associated with AHC.
Van Hillegondsberg and Michaelis reported using verapamil 6.6 mg/kg/day in three doses in 5-year-old pediatric patients.64 They observed a reduction in the frequency, intensity, and duration of episodes, with a 6-month marked abatement of severe debilitating plegic episodes in a significant number of individuals.64
Two AHC patients were treated by Samanta and Ramakrishnaiah using intravenous immunoglobulin (IVIG) infusion.65 A 2-year-old boy receiving IVIG infusion for 10 months was able to be seizure-free for 2 years after starting treatment, but unfortunately hemiplegic episodes recurred. An 8-year-old girl received periodic IVIG infusion for 4 years, which resulted in remission of paroxysmal events during the first 16 months of treatment.65
Utilizing Sleep as a Management Strategy Several studies have identified triggers in around half of the patients with AHC, such as exposure to cold, stress, fatigue, bathing, temperature fluctuations, and respiratory infections. 66 However, excessive avoidance of stressful factors might significantly compromise the quality of life and increase the incidence of psychiatric conditions such as anxiety, depression, and compulsive behaviors. Handling acute episodes is challenging, and developing coping strategies is essential, which can be facilitated by cognitive behavioral therapy. Early sleep induction is recommended due to its consistent alleviation of motor symptoms. 67 Methods include oral midazolam or melatonin administration. Though these are not officially approved for this purpose, they can be used on a case-by-case basis. 59 Also, pain relief using paracetamol or ibuprofen might mitigate the distress during attacks. 56
Addressing Epilepsy Epilepsy affects about half of the patients with AHC, often manifesting distinctly from AHC attacks. 68 In this context, there are reports of status epilepticus in individuals with AHC. However, there is no universally accepted drug for preventing AHC episodes, and epilepsy is usually suboptimally treated. 69 Motor symptoms persist between attacks and require assessment and management that takes into account mobility, dyspraxia, dystonia, chorea, tremors, weakness, and spasticity. 70 Surgical intervention remains uncommon due to uncertainties about its effectiveness during acute episodes.
Vagus nerve stimulation was studied in individuals with AHC and epilepsy. Helseth et al. revealed a more than 80% reduction in seizure frequency and 60% reduction in hemiplegic and dystonic episodes. The nerve stimulation parameters were not provided, but the adverse events were mild, with a low increase in the apnea-hypopnea index.71
Topiramate was the most commonly reported antiseizure medication with AHC. A common pathophysiology between seizures and AHC has been hypothesized due to the therapeutic benefits of topiramate in both of these conditions. Other antiseizure medications used were ethosuximide, felbamate, phenobarbital, phenytoin, and valproic acid. Interestingly, no antiseizure effect in the ATP was observed in different studies.68
Multidisciplinary Approach The AHC disorder can be differentiated from other movement disorders in several aspects, mainly due to the multifactorial involvement of several aspects of daily life. Therefore, programs with patients with AHC would benefit from multidisciplinary teams because they can optimize symptomatic and preventive management of this syndrome ( Table 5). 12, 19, 59, 68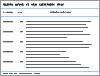 | Table 5 Management of Disorders Commonly Found in Patients with Alternating Hemiplegia of Childhood. |
One example of the significance of multidisciplinary evaluation is the nutritional deficiencies in this group of individuals. Most AHC-affected children tend to be small and underweight due to reduced calorie intake during episodes.43 Nutritional consultation by a dietitian with observation of daily calorie intake is beneficial for the continuous development of the patient. Also, if necessary, gastrostomy should be considered if conservative management is not effective, mainly in those individuals with significant oropharyngeal dysfunction due to a high risk of aspiration pneumonia.
A formal neuropsychological assessment is another critical aspect that is sometimes neglected in individuals with rare disorders. Board-certified neuropsychologists are recommended to perform the tests in every patient with AHC at the diagnosis, which should be repeated periodically. A significant number of patients with AHC will develop attention-deficit/hyperactivity disorder and mood disorder that sometimes can be difficult to distinguish and can be misdiagnosed as cognitive impairment.
Medications Used in AHC Treatment Several agents have already been attempted to manage AHC, and there are no disease-modifying therapies for this syndrome. However, the discovery of the ATP1A3 mutation in AHC in the last decade may significantly alter the treatment of this disorder in the subsequent years. Prophylactic agents are summarized in Table 6. 5, 33, 58, 62, 63, 72– 85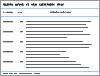 | Table 6 Medications Used in the Management of Alternating Hemiplegia of Childhood. |
|
Alternating hemiplegia of childhood is a multifaceted and varied disorder that goes beyond hemiplegic episodes, encompassing a range of clinical dysfunctions that notably involve the autonomic nervous system and musculoskeletal system, often leading to seizures and cognitive impairments. Patients with AHC exhibit a spectrum of symptoms, varying from mild to severe manifestations, alongside associated disorders.
The multi-level dysfunctions can significantly impact the long-term well-being of children affected by AHC. While hemiplegic episodes tend to decrease with age, a recent study by Cordani et al. reported epileptic seizures in 62% of the patients with AHC.1,8,29 Also, the authors observed that seizures can potentially play a detrimental role in the pathophysiological progression, particularly in individuals with drug-resistant epilepsy.29 Although there is no evidence suggesting AHC affects life expectancy, patients may experience complications related to cognitive impairment and oropharyngeal dysfunction, leading to life-threatening aspiration. Pavone et al. revealed that patients with persistent neurological findings compared to paroxysmal episodes, in which migraine, fine and gross motor dysfunction, walking disturbances, speech problems, and cognitive impairments are found, will more commonly have these features throughout their lives.86
In sporadic AHC cases, the clinical course tends to be more severe than in familial cases.7 The prognosis significantly depends on the age of onset, especially when hemiplegic spells begin early.87 Children displaying neonatal-onset symptoms experience severe developmental impacts, mainly when recurrent convulsive status epilepticus occurs, leading to psychomotor deterioration.43 While some children affected by motor dysfunctions require wheelchair assistance, others achieve independence in adulthood.20 As individuals grow older, hemiplegic episodes, abnormal ocular movements, and hypotonia become less frequent and less severe. The natural progression of AHC is often characterized by unpredictable relapses and remissions, not consistent until adulthood, suggesting the potential existence of different AHC subtypes.2 |
AHC AND ATP1A3-RELATED DISORDERS
Mutations within ATP1A3 are prevalent in AHC cases, accounting for approximately 78%, as noted in one study,36 and rising to 92% according to a recent Italian investigation.29 Besides AHC, these mutations have associations with other clinical conditions, forming a spectrum known as ATP1A3-related disorders (Figure 5).88 This spectrum encompasses distinct entities like AHC, RPD, and CAPOS syndromes.35,47 Additionally, EIEE, relapsing encephalopathy with cerebellar ataxia, and childhood rapid-onset ataxia have similar clinical manifestations and should be considered part of this spectrum. There are also reports of intermediate phenotypes or individuals showing atypical features not entirely fitting the classical signs of the disorders mentioned above.39,49,89
Fever-induced Paroxysmal Weakness and Encephalopathy Patients with the ATP1A3 R756H mutation experience childhood onset with sporadic fever-induced episodes causing encephalopathy and weakness. Long-term outcomes vary, ranging from mild motor issues to dysphagia. Some exhibit symptoms like dysarthria, cognitive problems, motor apraxia, and gait difficulties. 90
Slowly Progressive Cerebellar Ataxia Without Paroxysms Two patients carrying different ATP1A3 variants showed slow, continuous cerebellar ataxia without episodic symptoms. Magnetic resonance imaging (MRI) scans revealed mild cerebellar cortical atrophy in both cases. 91
Childhood-onset Schizophrenia/Autistic Spectrum Disorder Cases of childhood-onset schizophrenia (linked to ATP1A3 mutations) exhibit symptoms such as selective mutism, severe behavioral disturbances, aggression, auditory hallucinations, and mild motor delay. Some patients also have autistic spectrum disorder alongside these clinical symptoms. 92, 93
Paroxysmal Dyskinesia Cases of hyperkinetic movements triggered by various factors involving painful postures lasting minutes to hours were reported. Genetic analysis revealed ATP1A3 mutations linked to these symptoms. Another family presented exercise-induced dystonia without plegic attacks. 24, 94
Cerebral Palsy/Spastic Paraparesis Several cases with ATP1A3 mutations showcased symptoms like spastic diplegia, developmental delay, epilepsy, and episodic neurological deterioration. One case exhibited static encephalopathy, microcephaly, and dystonia. 95
Dystonia, Dysmorphism, Encephalopathy, MRI Abnormalities, and No Hemiplegia Patients with ATP1A3 variants presented with dystonia, seizures, and facial dysmorphism; cerebellar abnormalities were detected through MRI scans. Symptoms varied among patients, including hypotonia, tremors, nystagmus, and epileptic seizures. 96 However, no hemiplegia was reported in this subgroup of individuals.
Congenital Hydrocephalus and Other Brain Abnormalities Reports in the literature highlighted cases of severe congenital hydrocephalus, Chiari malformation, schizencephaly, and corpus callosum dysgenesis linked to ATP1A3 mutations. Additionally, patients with heterozygous ATP1A2/ ATP1A3 mutations showed developmental and epileptic encephalopathy, often associated with cortical developmental malformations leading to early lethality in some cases. 97, 98 |
Animal models are valuable tools for studying ATP1A3 disorders, especially due to the high degree of similarity between the genomics of humans and animal models (Table 7).4,99–104 Specifically, the amino acid sequence similarity between the mouse and human Na+/K+-ATPase alpha-3 subunits is approximately 99%.105 The first animal model for ATP1A3-related disorders was developed by Moseley et al.106 This mouse model was based on a point mutation in ATP1A3 intron 4 (targeted knock-in), which was subsequently replicated by Kirshenbaum et al. and DeAndrade et al.99,107 The animal model ATP1A3tm/1Ling/+ has increased ambulation in the open field, increased methamphetamine response, and increased hidden latency in the water maze. However, it does not develop spontaneous seizures.108 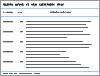 | Table 7 Summary of Pre-clinical Mouse Models of ATP1A3-related Disorders. |
Other models of ATP-related disorders present in the literature are drosophilas and zebrafish. Interestingly, all the flies with a mutation in human Na+/K+-ATPase alpha-3 subunits (Ala588Thr, Gly528Ser, Gly744Ser, Pro262Leu, Ser201Leu, Ser348Thr) showed mechanical stress-induced paralysis. However, only three had temperature-sensitive paralysis (Gly744Ser, Asp981Asn, and Glu982Lys).105 Zebrafish models are more challenging to analyze because their ATP1A3 comprises two orthologues, ATP1A3a and ATP1A3b. Also, it is suggested that both α3 paralogues are needed for embryonic motility.109 |
Diagnostic criteria with specific tests are urgently needed to help differentiate AHC from other ATP1A3-related disorders. The present diagnostic criteria format based only on the clinical criteria leads to significant overlap among the different diseases associated with the ATP1A3 mutations, as shown in Figure 5.
Another area that should be studied is the management of AHC. There are only retrospective studies with flunarizine, and isolated case reports with some medications. There is an urgent need for medications that can change the course of this condition, decreasing the number of paroxysmal attacks and promoting a better quality of life. |
Alternating hemiplegia of childhood is a complex and diverse disorder characterized primarily by hemiplegic episodes, varying widely in frequency and intensity. These episodes commonly coincide with epilepsy, developmental delay, movement disorders, and autonomic nervous system dysfunction. Most AHC cases are associated with ATP1A3-related disorders, encompassing RDP, CAPOS syndrome, EIEE, childhood rapid-onset ataxia, and relapsing encephalopathy with cerebellar ataxia. |
AHC |
alternating hemiplegia of childhood |
CAPOS |
cerebellar ataxia, areflexia, pes cavus, optic atrophy, and sensorineural hearing loss |
EIEE |
early infantile epileptic encephalopathy |
IVIG |
intravenous immunoglobulin |
MRI |
magnetic resonance imaging |
RDP |
rapid-onset dystonia-parkinsonism. |
|
|
2. Fons C, Campistol J, Panagiotakaki E, et al. Alternating hemiplegia of childhood: metabolic studies in the largest European series of patients. Eur J Paediatr Neurol 2012;16:10–14. https://doi.org/10.1016/j.ejpn.2011.08.006. 3. Hoei-Hansen CE, Dali CÍ, Lyngbye TJB, Duno M, Uldall P. Alternating hemiplegia of childhood in Denmark: clinical manifestations and ATP1A3 mutation status. Eur J Paediatr Neurol 2014;18:50–4. https://doi.org/10.1016/j.ejpn.2013.08.007. 4. Hunanyan AS, Fainberg NA, Linabarger M, et al. Knock-in mouse model of alternating hemiplegia of childhood: behavioral and electrophysiologic characterization. Epilepsia 2015;56:82–93. https://doi.org/10.1111/epi.12878. 5. Sweney MT, Silver K, Gerard-Blanluet M, et al. Alternating hemiplegia of childhood: early characteristics and evolution of a neurodevelopmental syndrome. Pediatrics 2009;123:e534–41. https://doi.org/10.1542/peds.2008-2027. 8. Incorpora G, Pavone P, Polizzi A, et al. An 11-year follow-up study of neonatal-onset, bath-induced alternating hemiplegia of childhood in twins. J Child Neurol 2012;27:657–62. https://doi.org/10.1177/0883073811436249. 10. Aicardi J, Bourgeois M, Goutieres F. Alternating Hemiplegia of Childhood: Clinical Findings and Diagnostic Criteria. In: Andermann F, Aicardi J, Vigevano F, eds. Alternating Hemiplegia of Childhood. New York: Raven Press; 1995:3–18. 11. Bourgeois M. Alternating Hemiplegia of Childhood. A Report of 29 Cases and a Review of the Literature. In: Arzimanoglu A, Goutières F, eds. Trends in Child Neurology. London: John Libbey Eurotext; 1996: 163–8. 13. Bursztyn J, Mikaeloff Y, Kaminska A, et al. [Alternating hemiplegia of childhoood and oculomotor anomalies]. J Fr Ophtalmol 2000;23:161–4. [Article in French] PMID: 10705111. 14. Silver K, Andermann F. Alternating Hemiplegia of Childhood: The Natural History of the Disorder in a Group of 10 Patients. In: Andermann F, Aicardi J, Vigevano F, eds. Alternating Hemiplegia of Childhood. New York: Raven Press; 1995:19–28. 16. Fusco L, Vigevano F. Alternating Hemiplegia of Childhood: Clinical Findings during Attacks. In: Andermann F, Aicardi J, Vigevano F, eds. Alternating Hemiplegia of Childhood. New York: Raven Press; 1995:29–41. 18. Bøttger P, Tracz Z, Heuck A, Nissen P, Romero-Ramos M, Lykke-Hartmann K. Distribution of Na/K-ATPase alpha 3 isoform, a sodium-potassium P-type pump associated with rapid-onset of dystonia parkinsonism (RDP) in the adult mouse brain. J Comp Neurol 2011;519:376–404. https://doi.org/10.1002/cne.22524. 19. Jasien JM, Bonner M, D’alli R, et al. Cognitive, adaptive, and behavioral profiles and management of alternating hemiplegia of childhood. Dev Med Child Neurol 2019;61:547–54. https://doi.org/10.1111/dmcn.14077. 21. Capuano A, Garone G, Tiralongo G, Graziola F. Alternating hemiplegia of childhood: understanding the genotype-phenotype relationship of ATP1A3 variations. Appl Clin Genet 2020;13:71–81. https://doi.org/10.2147/TACG.S210325. 23. Viollet L, Glusman G, Murphy KJ, et al. Alternating hemiplegia of childhood: retrospective genetic study and genotype-phenotype correlations in 187 subjects from the US AHCF registry. PLoS One 2015;10: e0127045. https://doi.org/10.1371/journal.pone.0127045. 24. Roubergue A, Roze E, Vuillaumier-Barrot S, et al. The multiple faces of the ATP1A3-related dystonic movement disorder. Mov Disord 2013;28:1457–9. https://doi.org/10.1002/mds.25396. 25. Boelman C, Lagman-Bartolome AM, MacGregor DL, McCabe J, Logan WJ, Minassian BA. Identical ATP1A3 mutation causes alternating hemiplegia of childhood and rapid-onset dystonia parkinsonism phenotypes. Pediatr Neurol 2014;51:850–3. https://doi.org/10.1016/j.pediatrneurol.2014.08.015. 26. Kanavakis E, Xaidara A, Papathanasiou-Klontza D, Papadimitriou A, Velentza S, Youroukos S. Alternating hemiplegia of childhood: a syndrome inherited with an autosomal dominant trait. Dev Med Child Neurol 2003;45:833–6. https://doi.org/10.1017/s0012162203001543. 28. Panagiotakaki E, De Grandis E, Stagnaro M, et al. Clinical profile of patients with ATP1A3 mutations in alternating hemiplegia of childhood—a study of 155 patients. Orphanet J Rare Dis 2015;10:123. https://doi.org/10.1186/s13023-015-0335-5. 29. Cordani R, Stagnaro M, Pisciotta L, et al. Alternating hemiplegia of childhood: genotype-phenotype correlations in a cohort of 39 Italian patients. Front Neurol 2021;12:658451. https://doi.org/10.3389/fneur.2021.658451. 30. Gurrieri F, Tiziano FD, Zampino G, Neri G. Recognizable facial features in patients with alternating hemiplegia of childhood. Am J Med Genet A 2016; 170:2698–705. https://doi.org/10.1002/ajmg.a.37808. 33. Vila-Pueyo M, Pons R, Raspall-Chaure M, et al. Clinical and genetic analysis in alternating hemiplegia of childhood: ten new patients from Southern Europe. J Neurol Sci 2014;344:37–42. https://doi.org/10.1016/j.jns.2014.06.014. 34. Ishii A, Saito Y, Mitsui J, et al. Identification of ATP1A3 mutations by exome sequencing as the cause of alternating hemiplegia of childhood in Japanese patients. PLoS One 2013;8:e56120. https://doi.org/10.1371/journal.pone.0056120. 35. Rosewich H, Thiele H, Ohlenbusch A, et al. Heterozygous de-novo mutations in ATP1A3 in patients with alternating hemiplegia of childhood: a whole-exome sequencing gene-identification study. Lancet Neurol 2012;11:764–73. https://doi.org/10.1016/S1474-4422(12)70182-5. 36. Heinzen EL, Swoboda KJ, Hitomi Y, et al. De novo mutations in ATP1A3 cause alternating hemiplegia of childhood. Nat Genet 2012;44:1030–4. https://doi.org/10.1038/ng.2358. 38. Holm TH, Isaksen TJ, Glerup S, et al. Cognitive deficits caused by a disease-mutation in the α3 Na(+)/K(+)-ATPase isoform. Sci Rep 2016;6:31972. https://doi.org/10.1038/srep31972. 39. Brashear A, Sweadner KJ, Cook JF, Swoboda KJ, Ozelius L. ATP1A3-related Neurologic Disorders. In: Adam MP, Feldman J, Mirzaa GM, et al., eds. GeneReviews(®). Seattle, WA: University of Washington, Seattle; 1993. https://www.ncbi.nlm.nih.gov/books/NBK1115/. 40. Verret S, Steele JC. Alternating hemiplegia in childhood: a report of eight patients with complicated migraine beginning in infancy. Pediatrics 1971;47:675–80. https://doi.org/10.1542/peds.47.4.675. 41. Guey S, Tournier-Lasserve E, Hervé D, Kossorotoff M. Moyamoya disease and syndromes: from genetics to clinical management. Appl Clin Genet 2015;8:49–68. https://doi.org/10.2147/TACG.S42772. 43. Panagiotakaki E, Gobbi G, Neville B, et al. Evidence of a non-progressive course of alternating hemiplegia of childhood: study of a large cohort of children and adults. Brain 2010;133:3598–610. https://doi.org/10.1093/brain/awq295. 46. Algahtani H, Ibrahim B, Shirah B, Aldarmahi A, Abdullah A. More than a decade of misdiagnosis of alternating hemiplegia of childhood with catastrophic outcome. Case Rep Med 2017;2017:5769837. https://doi.org/10.1155/2017/5769837. 48. Sabouraud P, Riquet A, Spitz M-A, et al. Relapsing encephalopathy with cerebellar ataxia are caused by variants involving p.Arg756 in ATP1A3. Eur J Paediatr Neurol 2019;23:448–55. https://doi.org/10.1016/j.ejpn.2019.02.004. 50. Swoboda KJ, Kanavakis E, Xaidara A, et al. Alternating hemiplegia of childhood or familial hemiplegic migraine? A novel ATP1A2 mutation. Ann Neurol 2004;55:884–7. https://doi.org/10.1002/ana.20134. 53. Villéga F, Picard F, Espil-Taris C, Husson M, Michel V, Pedespan J-M. Benign nocturnal alternating hemiplegia of childhood: two cases with positive evolution. Brain Dev 2011;33:525–9. https://doi.org/10.1016/j.braindev.2010.08.008. 54. Maas RPPWM, Kamsteeg E-J, Mangano S, et al. Benign nocturnal alternating hemiplegia of childhood: a clinical and nomenclatural reappraisal. Eur J Paediatr Neurol 2018;22:1110–17. https://doi.org/10.1016/j.ejpn.2018.07.012. 55. Thomsen LL, Eriksen MK, Roemer SF, Andersen I, Olesen J, Russell MB. A population-based study of familial hemiplegic migraine suggests revised diagnostic criteria. Brain 2002;125:1379–91. https://doi.org/10.1093/brain/awf132. 57. Pavone P, Pappalardo XG, Incorpora G, et al. Long-term follow-up and novel genotype-phenotype analysis of monozygotic twins with ATP1A3 mutation in alternating hemiplegia of childhood-2. Eur J Med Genet 2020;63:103957. https://doi.org/10.1016/j.ejmg.2020.103957. 60. Bourgeois M, Aicardi J, Andermann F, Aicardi J, Vigevano F. The Treatment of Alternating Hemiplegia in Childhood with Flunarizine. In: Andermann F, Aicardi J, Vigevano F, eds. Alternating Hemiplegia of Childhood. New York: Raven Press; 1994:191–3. 61. Sakuragawa N. The Treatment of Alternating Hemiplegia of Childhood in Japan. In: Andermann F, Aicardi J, Vigevano F, eds. Alternating Hemiplegia of Childhood. New York: Raven Press; 1995:199. 66. Guevara-Campos J, González-de Guevara L, Urbáez-Cano J, Tinedo R, Villamizar M, Rojas L. [Alternating hemiplegia of childhood treated as epilepsy. Two new cases]. Rev Neurol 2005;40:351–3. [Article in Spanish] PMID: 15795871. 67. Zhang Y, Sun W, Qin J, Jiang Y, Wu X. [Clinical characteristics of alternating hemiplegia of childhood in 13 patients]. Zhonghua Er Ke Za Zhi 2003;41:680–3. [Article in Chinese] PMID: 14733811. 73. Sone K, Oguni H, Katsumori H, Funatsuka M, Tanaka T, Osawa M. Successful trial of amantadine hydrochloride for two patients with alternating hemiplegia of childhood. Neuropediatrics 2000;31:307–9. https://doi.org/10.1055/s-2000-12945. 79. Roubergue A, Philibert B, Gautier A, et al. Excellent response to a ketogenic diet in a patient with alternating hemiplegia of childhood. JIMD Rep 2015; 15:7–12. https://doi.org/10.1007/8904_2013_292. 81. Korinthenberg R. Is infantile alternating hemiplegia mediated by glutamate toxicity and can it be treated with memantine? Neuropediatrics 1996;27:277–8. https://doi.org/10.1055/s-2007-973779. 82. Ju J, Hirose S, Shi X-Y, Ishii A, Hu L-Y, Zou L-P. Treatment with oral ATP decreases alternating hemiplegia of childhood with de novo ATP1A3 Mutation. Orphanet J Rare Dis 2016;11:55. https://doi.org/10.1186/s13023-016-0438-7. 83. Chi L, Zhao X, Liu X, Jiang W, Chi Z, Wang S. Alternating hemiplegia of childhood in chinese following long-term treatment with flunarizine or topiramate. Int J Neurosci 2012;122:506–10. https://doi.org/10.3109/00207454.2012.683216. 85. Hainque E, Caillet S, Leroy S, et al. A randomized, controlled, double-blind, crossover trial of triheptanoin in alternating hemiplegia of childhood. Orphanet J Rare Dis 2017;12:160. https://doi.org/10.1186/s13023-017-0713-2. 86. Pavone P, Pappalardo XG, Ruggieri M, Falsaperla R, Parano E. Alternating hemiplegia of childhood: a distinct clinical entity and ATP1A3-related disorders: a narrative review. Medicine (Baltimore) 2022;101: e29413. https://doi.org/10.1097/MD.0000000000029413. 89. Lazarov E, Hillebrand M, Schröder S, et al. Comparative analysis of alternating hemiplegia of childhood and rapid-onset dystonia-parkinsonism ATP1A3 mutations reveals functional deficits, which do not correlate with disease severity. Neurobiol Dis 2020; 143:105012. https://doi.org/10.1016/j.nbd.2020.105012. 91. Sasaki M, Sumitomo N, Shimizu-Motohashi Y, et al. ATP1A3 variants and slowly progressive cerebellar ataxia without paroxysmal or episodic symptoms in children. Dev Med Child Neurol 2021;63:111–15. https://doi.org/10.1111/dmcn.14666. 92. Smedemark-Margulies N, Brownstein CA, Vargas S, et al. A novel de novo mutation in ATP1A3 and childhood-onset schizophrenia. Cold Spring Harb Mol Case Stud 2016;2:a001008. https://doi.org/10.1101/mcs.a001008. 93. Chaumette B, Ferrafiat V, Ambalavanan A, et al. Missense variants in ATP1A3 and FXYD gene family are associated with childhood-onset schizophrenia. Mol Psychiatry 2020;25:821–30. https://doi.org/10.1038/s41380-018-0103-8. 94. Zúñiga-Ramírez C, Kramis-Hollands M, Mercado-Pimentel R, et al. Generalized dystonia and paroxysmal dystonic attacks due to a novel ATP1A3 variant. Tremor Other Hyperkinet Mov (N Y) 2019;2019:9. https://doi.org/10.7916/tohm.v0.723. 97. Allocco AA, Jin SC, Duy PQ, et al. Recessive inheritance of congenital hydrocephalus with other structural brain abnormalities caused by compound heterozygous mutations in ATP1A3. Front Cell Neurosci 2019;13:425. https://doi.org/10.3389/fncel.2019.00425. 98. Vetro A, Nielsen HN, Holm R, et al. ATP1A2- and ATP1A3-associated early profound epileptic encephalopathy and polymicrogyria. Brain 2021;144:1435–50. https://doi.org/10.1093/brain/awab052. 99. Kirshenbaum GS, Clapcote SJ, Duffy S, et al. Mania-like behavior induced by genetic dysfunction of the neuron-specific Na+,K+-ATPase α3 sodium pump. Proc Natl Acad Sci U S A 2011;108:18144–9. https://doi.org/10.1073/pnas.1108416108. 101. Holm TH, Lykke-Hartmann K. Insights into the pathology of the α3 Na(+)/K(+)-ATPase ion pump in neurological disorders; lessons from animal models. Front Physiol 2016;7:209. https://doi.org/10.3389/fphys.2016.00209. 102. Clapcote SJ, Duffy S, Xie G, et al. Mutation I810N in the alpha3 isoform of Na+,K+-ATPase causes impairments in the sodium pump and hyperexcitability in the CNS. Proc Natl Acad Sci U S A 2009;106:14085–90. https://doi.org/10.1073/pnas.0904817106. 107. DeAndrade MP, Yokoi F, van Groen T, Lingrel JB, Li Y. Characterization of ATP1A3 mutant mice as a model of rapid-onset dystonia with parkinsonism. Behav Brain Res 2011;216:659–65. https://doi.org/10.1016/j.bbr.2010.09.009. 109. Doğanli C, Beck HC, Ribera AB, Oxvig C, Lykke-Hartmann K. α3Na+/K+-ATPase deficiency causes brain ventricle dilation and abrupt embryonic motility in zebrafish. J Biol Chem 2013;288:8862–74. https://doi.org/10.1074/jbc.M112.421529. |